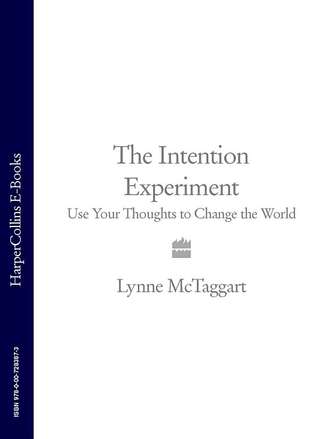
Полная версия
The Intention Experiment: Use Your Thoughts to Change the World
These back-and-forth passes, which rise to an extraordinarily large ground state of energy, are known collectively as the Zero Point Field. The field is called ‘zero point’ because even at temperatures of absolute zero, when all matter theoretically should stop moving, these tiny fluctuations are still detectable. Even at the coldest place in the universe, subatomic matter never comes to rest, but carries on this little energy tango.16
The energy generated by every one of these exchanges between particles is unimaginably tiny – about half a photon’s worth. However, if all exchanges between all subatomic particles in the universe were to be added up, it would produce an inexhaustible supply of energy of unfathomable proportions, exceeding all energy in matter by a factor of 1040, or 1 followed by 40 zeros.17 Richard Feynman himself once remarked that the energy in a cubic metre of space was enough to boil all the oceans of the world.18
After the discoveries of Heisenberg about Zero Point energy, most conventional physicists have subtracted the figures symbolizing Zero Point energy from their equations. They assumed that, because the Zero Point Field was ever present in matter, it did not change anything and so could be safely ‘renormalized’ away. However, in 1973, when trying to work out an alternative to fossil fuel during the petrol crisis, American physicist Hal Puthoff, inspired by the Russian Andrei Sakharov, began trying to figure out how to harness the teeming energy of empty space for transport on earth and to distant galaxies. Puthoff spent more than 30 years examining the Zero Point Field. With some colleagues, he had proved that this constant energy exchange of all subatomic matter with the Zero Point Field accounts for the stability of the hydrogen atom, and, by implication, the stability of all matter.19 Remove the Zero Point Field and all matter would collapse in on itself. He also demonstrated that Zero Point energy is responsible for two basic properties of mass: inertia and gravity.20 Puthoff also worked on a multimillion-dollar project funded by Lockheed Martin and a variety of American universities, to develop Zero Point energy for space travel – a programme that finally went public in 2006.
Many strange properties of the quantum world, like uncertainty or entanglement, could be explained if you factored in the constant interaction of all quantum particles with the Zero Point Field. To Puthoff, science’s understanding of the nature of entanglement was analogous to two sticks stuck in the sand at the edge of the ocean, about to be hit by a huge wave. If they both were knocked over, and you did not know about the wave, you would think that one stick was affecting the other and call it a non-local effect. The constant interaction of quantum particles with the Zero Point Field might be the underlying mechanism for non-local effects between particles, allowing one particle to be in touch with every other particle at any moment.21
Benni Reznik’s work in Israel with the Zero Point Field and entanglement began mathematically with a central question: what would happen to a hypothetical pair of probes interacting with the Zero Point Field? According to his calculations, once they began interacting with the Zero Point Field, the probes would begin talking to each other and ultimately become entangled.22
If all matter in the universe were interacting with the Zero Point Field, it meant, quite simply, that all matter was interconnected and potentially entangled throughout the cosmos through quantum waves.23 And if we and all of empty space are a mass of entanglement, we must be establishing invisible connections with things at a distance to ourselves. Acknowledging the existence of the Zero Point Field and entanglement offers a ready mechanism for why signals being generated by the power of thought can be picked up by someone else many miles away.
* * * Sai Ghosh had proved that non-locality existed in the large building blocks of matter and the other scientists proved that all matter in the universe was, in a sense, a satellite of a large central energy field. But how could matter be affected by this connection? The central assumption of all of classical physics is that large material things in the universe are set pieces, a fait accompli of manufacture. How can they possibly be changed?
Vedral had an opportunity to examine this question when he was invited to work with the renowned quantum physicist Anton Zeilinger. Zeilinger’s Institute for Experimental Physics lab at the University of Vienna was at the very frontier of some of the most exotic research into the nature of quantum properties. Zeilinger himself was profoundly dissatisfied with the current scientific explanation of nature, and he had passed on that dissatisfaction and the quest to resolve it to his students.
In a flamboyant gesture, Zeilinger and his team had entangled a pair of photons from beneath the River Danube. They had set up a quantum channel via a glass fibre and run it across the river bed of the Danube. In his lab, Zeilinger liked to refer to individual photons as Alice and Bob, and sometimes, if he needed a third photon, Carol or Charlie. Alice and Bob, separated by 600 metres of river and nowhere in sight of each other, maintained a non-local connection.24
Zeilinger was particularly interested in superposition, and the implications of the Copenhagen Interpretation – that subatomic particles exist only in a state of potential. Could objects, and not simply the subatomic particles that compose them, he wondered, exist in this hall-of-mirrors state? To test this question, Zeilinger employed a piece of equipment called a Talbot Lau interferometer, developed by some colleagues at MIT, using a variation on the famous double-slit experiment of Thomas Young, a British physicist of the nineteenth century. In Young’s experiment, a beam of pure light is sent through a single hole, or slit, in a piece of cardboard, then passes through a second screen with two holes before finally arriving at a third, blank screen.
When two waves are in phase (that is, peaking and troughing at the same time), and bump into each other – technically called ‘interference’ – the combined intensity of the waves is greater than each individual amplitude. The signal gets stronger. This amounts to an imprinting or exchange of information, called ‘constructive interference’. If one is peaking when the other troughs, they tend to cancel each other out – called ‘destructive interference’. With constructive interference, when all the waves are wiggling in synch, the light will get brighter; destructive interference will cancel out the light and result in complete darkness.
In the experiment, the light passing through the two holes forms a zebra pattern of alternating dark and light bands on the final blank screen. If light were simply a series of particles, two of the brightest patches would appear directly behind the two holes of the second screen. However, the brightest portion of the pattern is halfway between the two holes, caused by the combined amplitude of those waves that most interfere with each other. From this pattern, Young was the first to realize that light beaming through the two holes spreads out in overlapping waves.
A modern variation of the experiment fires off single photons through the double slit. These single photons also produce zebra patterns on the screen, demonstrating that even single units of light travel as a smeared-out wave with a large sphere of influence.
Twentieth-century physicists went on to use Young’s experiment with other individual quantum particles, and held it up as proof that quantum physics had Through-the-Looking-Glass properties: quantum entities acted wavelike and travelled though both slits at once. Fire a stream of electrons at the triple screens, and you end up with the interference patterns of alternating light and dark patches, just as you do with a beam of light. Since you need at least two waves to create such interference patterns, the implication of the experiment is that the photon is somehow mysteriously able to travel through both slits at the same time and interfere with itself when it reunites.
The double-slit experiment encapsulates the central mystery of quantum physics – the idea that a subatomic particle is not a single seat but the entire stadium. It also demonstrates the principle that electrons, which exist in a hermetic quantum state, are ultimately unknowable. You could not identify something about a quantum entity without stopping the particle in its tracks, at which point it would collapse to a single point.
In Zeilinger’s adaptation of the slit experiment, using molecules instead of subatomic particles, the interferometer contained an array of slits in the first screen, and a grating of identical parallel slits in the second one, whose purpose was to diffract (or deflect) the molecules passing by. The third grating, turned perpendicular to the beam of molecules, acted as a scanning ‘mask’, with the ability to calculate the size of the waves of any of the molecules passing through, by means of a highly sensitive laser detector to locate the positions of the molecules and their interference patterns.
For the initial experiment, Zeilinger and his team carefully chose a batch of fullerene molecules, or ‘buckyballs’ made of 60 carbon atoms. At one nanometre apiece, these are the behemoths of the molecular world. They selected fullerene not only for its size but also for its neat arrangement, with a shape like a tiny symmetrical football.
It was a delicate operation. Zeilinger’s group had to work with just the right temperature; heating the molecules just a hair too much would cause them to disintegrate. Zeilinger heated the fullerenes to 900 K so they would create an intense molecular beam, then fired them through the first screen; they then passed through the second screen before making a pattern on the final screen. The results were unequivocal. Each molecule displayed the ability to create interference patterns with itself. Some of the largest units of physical matter had not ‘localized’ into their final state. Like a subatomic particle, these giant molecules had not yet gelled into anything real.
The Vienna team scouted out some other molecules that were double the size and oddly shaped to see if geometrically asymmetric molecules also demonstrated the same magical properties. They settled on gigantic fluorinated American football-shaped molecules of 70 carbon atoms and pancake-shaped tetraphenylporphyrin, a derivative of the biodye present in chlorophyll. At more than 100 atoms apiece, both of these entities are among the largest molecules on the planet. Again, each one created an interference pattern with itself.
Zeilinger’s group repeatedly demonstrated that the molecules could be two places at once, which meant that they remained in a state of superposition even at this large scale.25 They had proved the unthinkable: the largest components of physical matter and living things exist in a malleable state.26
Sai Ghosh didn’t often think about the implications of her discovery. She was content with the knowledge that her experiment had made a very nice paper, and might help along her career as an assistant professor involved in research into miniaturization, the direction she believed quantum mechanics was heading. Occasionally, she allowed herself to speculate that her crystal might have proved something important about the nature of the universe. But she was only a postgraduate student. What did she, after all, really know about how the world worked?
But to me, Ghosh’s research and Zeilinger’s work on the double-slit experiment represent two defining moments in modern physics. Ghosh’s experiments show that an invisible connection exists between the fundamental elements of matter, which is often so strong that it can override classical methods of influence, such as heat or a push. Zeilinger’s work demonstrated something even more astonishing. Large matter was neither something solid and stable nor something that necessarily behaved according to Newtonian rules. Molecules needed some other influence to settle them into a completed state of being.
Theirs were the first evidence that the peculiar properties of quantum physics do not simply occur at the quantum level with subatomic particles, but also in the world of visible matter. Molecules also exist in a state of pure potential, not a final actuality. Under certain circumstances, they escape Newtonian rules of force and display quantum non-local effects. The fact that something as large as a molecule can become entangled suggests that there are not two rule books – the physics of the large and the physics of the small – but only a single rule book for all of life.
These two experiments also hold the key to a science of intention – how thoughts are able to affect finished, solid matter. They suggest that the observer effect occurs not simply in the world of the quantum particle but also in the world of the everyday. Things no longer should be seen to exist in and of themselves but, like a quantum particle, only in relationship. Co-creation and influence may be a basic, inherent property of life. Our observation of every component in our world may help to determine its final state, which suggests that we are likely to be influencing every large thing we see around us. When we enter a crowded room, when we engage with our partners and our children, when we gaze up at the sky, we may be creating and even influencing at every moment. We can’t yet demonstrate this at normal temperatures; our equipment is still too crude. But we already have some preliminary proof: the physical world – matter itself – appears to be malleable, susceptible to influence from the outside.
Notes - Chapter 1: Mutable Matter
1. All personal information about Tom Rosenbaum and Sai Ghosh and their studies have been culled from multiple interviews conducted in February and March 2005.
2. This was the solution posed by Giorgio Parisi at Rome in 1979.
3. S. Ghosh et al., ‘Coherent spin oscillations in a disordered magnet’, Science, 2002; 296: 2195–8.
4. Once again, I am indebted to Danah Zohar for her easy-to-digest description of quantum non-locality, which appears in D. Zohar, The Quantum Self, London: Bloomsbury, 1991: 19–20.
5. A. Einstein, B. Podolsky and N. Rosen, ‘Can quantum-mechanical description of physical reality be considered complete?’ Physical Review, 1935; 47: 777–80.
6. A. Aspect et al., ‘Experimental tests of Bell’s inequalities using time-varying analyzers’, Physical Review Letters, 1982; 49: 1804–7; A. Aspect, ‘Bell’s inequality test: more ideal than ever’, Nature, 1999; 398: 189–90.
7. Science Fact: Scientists achieve ‘Star Trek’-like feat – The Associated Press, December 10, 1997, posted on CNN, http://edition.cnn.com/TECH/9712/10/beam. me. up. ap.
8. Non-locality was considered to be proven by Aspect et al.’ s experiments in Paris in 1982.
9. J. S. Bell, ‘On the Einstein-Poldolsky-Rosen paradox’, Physics, 1964; 1: 195–200.
10. S. Ghosh et al., ‘Entangled quantum state of magnetic dipoles’, Nature, 2003; 435: 48–51.
11. Details of Vedral’s views and experiments the result of multiple interviews, February, October and December 2005.
12. C. Arnesen et al., ‘Thermal and magnetic entanglement in the 1D Heisenberg Model’, Physical Review Letters, 2001; 87: 017901.
13. V. Vedral, ‘Entanglement hits the big time’, Nature, 2003; 425: 28–9.
14. T. Durt, interview with author, April 26, 2005.
15. B. Reznik, ‘Entanglement from the vacuum’, Foundations of Physics, 2003; 33: 167–76; Michael Brooks, ‘Entanglement: The weirdest link’, New Scientist, 2004; 181 (2440): 32.
16. John D. Barrow, The Book of Nothing, London: Jonathan Cape, 2000: 216.
17. Erwin Laszlo, The Interconnected Universe: Conceptual Foundations of Transdiscipinary Unified Theory, Singapore: World Scientific Publishing, 1995: 28.
18. A. C. Clarke, ‘When will the real space age begin?’ Ad Astra, May–June 1996; 13–15.
19. Harold Puthoff, ‘Ground state of hydrogen as a zero-point-fluctuation-determined state’, Physical Review D, 1987; 35: 3266.
20. B. Haisch, Alfonso Rueda and H. E. Puthoff, ‘Inertia as a zero-point-field Lorentz force’, Physical Review A, 1994; 49 (2): 678–94; Bernhard Haisch, Alfonso Rueda and H. E. Puthoff, ‘Physics of the zero-point field: implications for inertia, gravitation and mass’, Speculations in Science and Technology, 1997; 20: 99–114.
21. Various interviews with Hal Puthoff, 1999–2000.
22. Reznik, ‘Entanglement from the vacuum’, op. cit.
23. McTaggart, The Field, op. cit.: 35–6.
24. J. Resch et al., ‘Distributing entanglement and single photons through an intra-city, free-space quantum channel’, Optics Express, 2005; 13 (1): 202–9; R. Ursin et al., ‘Quantum teleportation across the Danube’, Nature, 2004; 430: 849.
25. M. Arndt et al., ‘Wave–particle duality of C60 molecules’, Nature, 1999; 401: 680–2; doi: 10.1038/44348.
26. A. Zeilinger, ‘Probing the limits of the quantum world’, Physics World, March 2005 (online journal: http://www.physicsweb.org/articles/world/18/3/5/1).
CHAPTER TWO
The Human Antenna
IN 1951, AT THE AGE OF SEVEN, Gary Schwartz made a remarkable discovery. He had been trying to get a good picture on the family’s television set. The recently acquired black and white Magnavox set encased behind the doors of its boxed walnut console fascinated him, not because of the people in the moving pictures so much as the means by which they arrived in his living room in the first place. The mechanisms of the relatively new invention remained a mystery, even to most adults. Television, like any other electrical gadget, was something the precocious child longed to take apart and understand. This passion had already found expression with the worn-out radios given to him by his grandfather. Ignatz Schwartz sold replacement tubes for televisions and radios in his drug store in Great Neck, Long Island, and those that were beyond repair were handed over to his grandson to disassemble. In a corner of Gary’s bedroom lay a mass of experimental debris – tubes, resistors and the carcasses of radios heaped on the cosmetic display racks he had borrowed from his grandfather – the first signs of what would become a lifelong fascination with electronics.
Gary knew that the way you twisted the rabbit-ear antenna on top of the television would determine the clarity of the picture. His father had explained that television sets were powered by something invisible, similar to radio waves, that flew through the air and were somehow translated into an image. Gary had even carried out some rudimentary experiments. When you stood somewhere between the antenna and the television, you could make the picture go away. When you touched the antenna in certain ways, you made the picture clearer.
One day, on a whim, Gary unscrewed the antenna and placed his finger on the screw where the cable had been. What had been a mass of squiggles and static noise on the screen suddenly coalesced into a perfect image. Even at that young age, he had understood that he had witnessed something extraordinary about human beings: his body was acting like a television antenna, a receiver of this invisible information. He tried the same experiment with a radio – substituting his finger for the antenna, and the same thing happened. Something in the makeup of a person was not unlike the rabbit ears that helped produce his television image. He too was a receiver of invisible information, with the ability to pick up signals transmitted across time and space.
Until he was 15, however, he could not visualize what these signals were made of. He had learned to play the electric guitar and had often wondered what unseen influences allowed the instrument to create different sounds. He could play the same note, middle C, and yet produce more of a treble or bass sound, depending on which way he turned the knob. How was it possible that a single note could sound so different? For a science project, he created multiple-track recordings of his music and then located a company in upstate New York that had equipment designed to analyse the frequency of sound. When he fed his recordings into the equipment, it quickly deconstructed the notes down to their essence. Each note registered as a batch of squiggles across the screen of the cathode-ray tube in front of him – a complex mix of hundreds of frequencies representing a blend of overtones that would subtly change when he turned the knob to treble or bass. He knew that these frequencies were waves, represented on the monitor as a sideways S, or sine curve, like a skipping rope held at both ends and wriggled, and that they had periodic oscillations, or fluctuations, similar to the waves on Long Island Sound. Every time he spoke, he knew he generated similar frequencies through his voice. He remembered his early television experiments and wondered whether a field of energy pulsated inside him and shared a kinship with sound waves.1
Gary’s childhood experiments may have been rudimentary, but he had already stumbled across the central mechanism of intention. Something in the quality of our thoughts was a constant transmission, not unlike a television station.
As an adult, Schwartz, still a bustling dynamo of enthusiasms, found an outlet in psychophysiology, then a fledgling study of the effect of the mind on the body. By the time he had accepted a post at the University of Arizona, which was known for encouraging freedom of research among its faculty, he had grown fascinated by biofeedback and the ways in which the mind could control blood pressure and a variety of illnesses – and the powerful physical effect of different types of thoughts.2
One weekend in 1994, at a conference on the relationship between love and energy, he sat in on a lecture by physicist Elmer Green, one of the pioneers of biofeedback. Green, like Schwartz, had grown interested in the energy being transmitted by the mind. To examine this more closely, he had decided to study remote healers and to determine whether they sent out more electrical energy than usual while in the process of healing.
Green reported in his lecture that he had built a room whose four walls and ceiling were entirely made of copper, and were attached to microvolt electroencephalogram (EEG) amplifiers – the kind used to measure the electrical activity in the brain. Ordinarily, an EEG amplifier is attached to a cap with imbedded electrodes, each of which records separate electrical discharges from different places in the brain. The cap is placed on a person’s head, and the electrical activity picked up is displayed on the amplifier. EEG amplifiers are extraordinarily sensitive, capable of picking up the most minute of effects – even one-millionth of a volt of electricity.
In remote healing, Green suspected that the signal produced was electrical and emanated from the healer’s hands. The copper wall acted like a giant antenna, magnifying the ability to detect the electricity from the healers and enabling Green to capture it from five directions.
He discovered that, whenever a healer sent healing, the EEG amplifier often recorded it as a huge surge of electrostatic charge, the same kind of the build up and discharge of electrons that occurs after you shuffle your feet along a new carpet and then touch a metal doorknob.3
In the early days of the copper wall experiment, Green had been faced with an enormous problem. Whenever a healer so much as wriggled a finger, patterns got recorded on an EEG amplifier. Green had had to work out a means of separating out the true effects of healing from this electrostatic noise. The only way to do so, as he saw it, was to have his healers remain perfectly still while they were sending out healing energy.