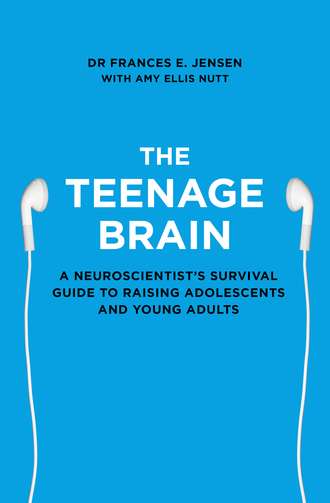
Полная версия
The Teenage Brain: A neuroscientist’s survival guide to raising adolescents and young adults
So what I have just told you is that brain areas for vision and body parts are compartmentalized in different places, but that they can shrink or grow relative to one another during development based on how much the senses are used. Structurally, the human brain is divided into four lobes: frontal (top front), parietal (top back), temporal (sides), and occipital (back). The brain sits on the brainstem, which connects to the spinal cord. In the rear of the brain, the cerebellum regulates motor patterning and coordination, and the occipital lobes house the visual cortex. The parietal lobes house association areas as well as the motor and sensory cortices (which include the homunculus in Figure 2). The temporal lobes include areas involved in the regulation of emotions and sexuality. Language is also located here, more specifically in the dominant hemisphere (the left temporal lobe for right-handed people and 85 percent of left-handed people, and the right temporal lobe for that small group of truly strong lefties). The frontal lobes sit most anteriorly and this area is concerned with executive function, judgment, insight, and impulse control. Importantly, as the brain matures from back to front in the teen years the frontal lobes are the least mature and the least connected compared with the other lobes.
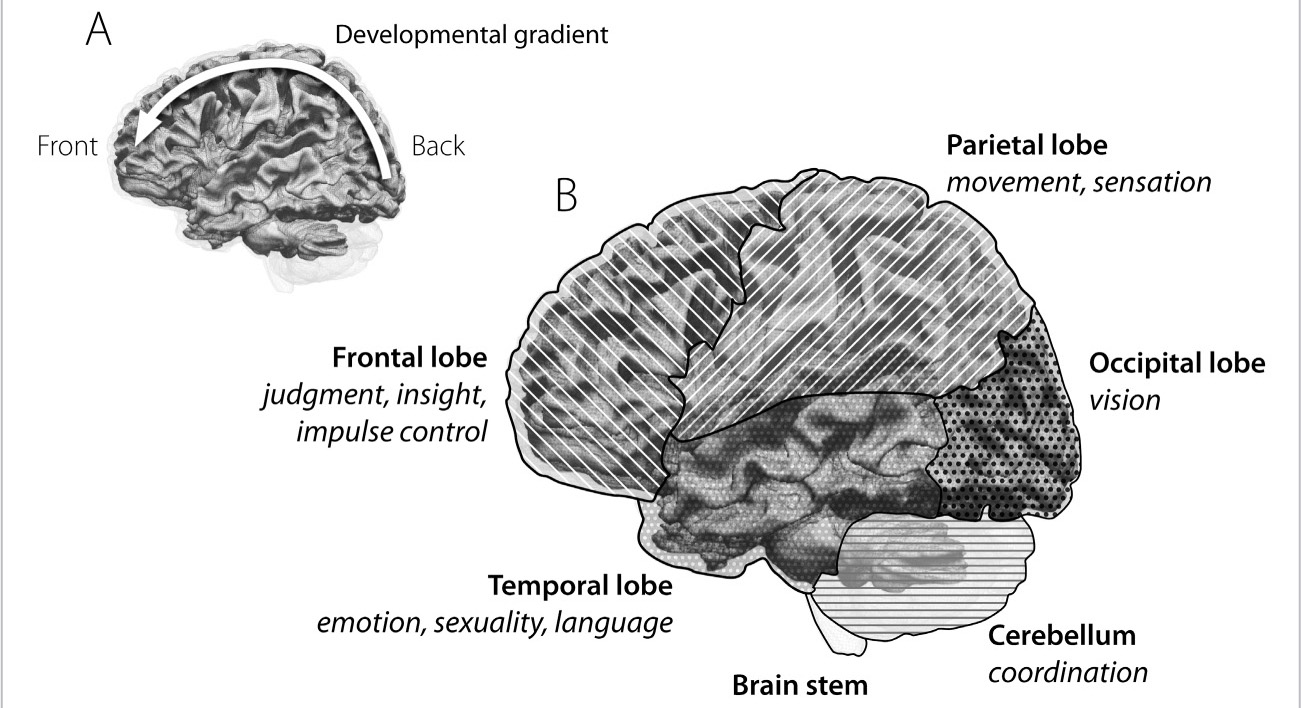
FIGURE 3. The Lobes of the Brain: A. The brain matures from the back to the front. B. The cortex of the brain can be divided into several main areas based on function.
The brain is divided into specialized regions for each of the senses. The area for hearing, or the auditory cortex, is in the temporal lobes; the visual cortex is in the occipital lobes; and the parietal lobes house movement and feeling in the motor and sensory cortices, respectively. Other parts of the brain have nothing to do with the senses, and the best example of this is the frontal lobes, which make up more than 40 percent of the human brain’s total volume—more than in any other animal species. The frontal lobes are the seat of our ability to generate insight, judgment, abstraction, and planning. They are the source of self-awareness and our ability to assess dangers and risk, so we use this area of the brain to choose a course of action wisely.
Hence, the frontal lobes are often said to house the “executive” function of the human brain. A chimpanzee’s frontal lobes come closest to the human’s in terms of size, but still make up only around 17 percent of its total brain volume. A dog’s frontal lobes make up just 7 percent of its brain. For other species, different brain structures are more important. Compared with humans, monkeys and chimpanzees have a much larger cerebellum, where control of physical coordination is honed. A dolphin’s auditory cortex is more advanced than a human’s, with a hearing range at least seven times that of a young adult. A dog has a billion olfactory cells in its brain compared with our measly twelve million. And the shark has special cells in its brain that help it detect electrical fields—not to navigate but to pick up electrical signals given off by the scantest of muscle movements in other fish as they try to hide from this deadly predator.
We humans don’t have a lot else going for us other than our wile and wit. Our competitive edge is our ingenuity, brains over brawn. This edge happens to take the longest time to develop, as the connectivity to and from the frontal lobes is the most complex and is the last to fully mature. This “executive function” thus develops slowly: we certainly are not born with it!
So in what order are these brain regions all connected to one another during childhood and adolescence? This could never have been learned before the advent of modern brain imaging. New forms of brain scans, called magnetic resonance imaging (MRI), not only can give us accurate pictures of the brain inside the skull but also can show us connections between different regions. Even better, a new kind of MRI, called the functional MRI, abbreviated fMRI, can actually show us what brain areas turn one another on. So we can actually see if areas that “fire” together are “wired” together. In the last decade, the National Institutes of Health conducted a major study to examine how brain regions activate one another over the first twenty-one years of life.
What they found was remarkable: the connectivity of the brain slowly moves from the back of the brain to the front. The very last places to “connect” are the frontal lobes (Figure 4). In fact, the teen brain is only about 80 percent of the way to maturity. That 20 percent gap, where the wiring is thinnest, is crucial and goes a long way toward explaining why teenagers behave in such puzzling ways—their mood swings, irritability, impulsiveness, and explosiveness; their inability to focus, to follow through, and to connect with adults; and their temptations to use drugs and alcohol and to engage in other risky behavior. When we think of ourselves as civilized, intelligent adults, we really have the frontal and prefrontal parts of the cortex to thank.
Because teens are not quite firing on all cylinders when it comes to the frontal lobes, we shouldn’t be surprised by the daily stories we hear and read about tragic mistakes and accidents involving adolescents. The process is not really done by the end of the teen years—and as a result the college years are still a vulnerable period. Recently a friend of mine told me about his son’s college classmate, Dan, an all-around great kid who’d rarely caused his parents to worry. He was popular, had been a star ice hockey player in high school, and was a finance major in college. Over the summer my friend’s son got a phone call from Dan’s mother. Dan had drowned the night before, she told him. He’d been out with friends, drinking, and sometime between three and four in the morning, on their way home, the group—there were eight of them—decided they wanted to cool off, so they stopped at the local tennis club. The club was closed, of course, but the locked gate didn’t stop them. All eight scaled the fence and jumped into the pool. It was only after they’d gotten home that someone said, “Where’s Dan?” Racing back to the club, they found their friend facedown in the water. The medical examiner listed the cause of death as accidental drowning due to “acute alcohol intoxication.” One of the news reports I read made me shake my head: “Police are asking kids and adults to think twice about potential dangers before taking any risks that could turn deadly.”
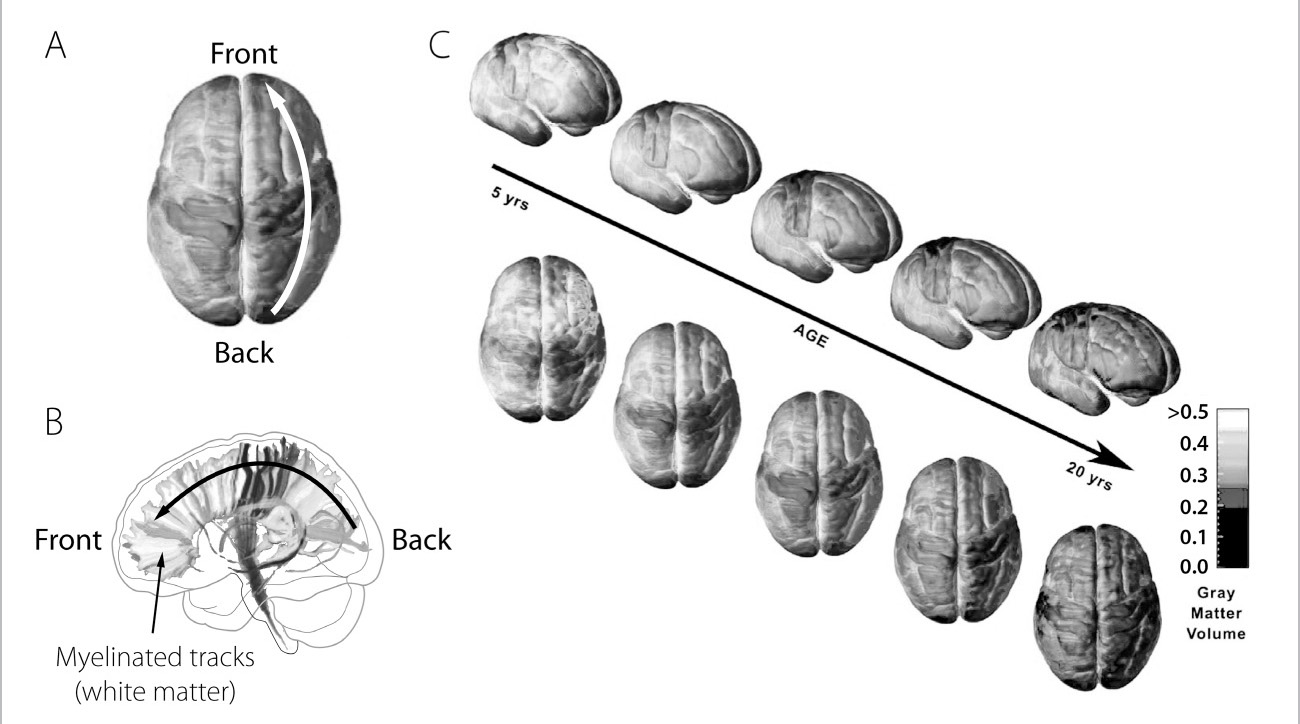
FIGURE 4. Maturing Brain: The Brain “Connects” from Back to Front: A. A functional MRI (fMRI) scan can map connectivity in the brain. Darker areas indicate greater connectivity. B. Myelination of white matter tracks cortex maturation from back to front; this is why the frontal lobes are the last to be connected. C. Serial connectivity scans reveal that frontal lobe connectivity is delayed until age twenty or older.
“Think twice.”
How many times have we all said this to our teenage sons and daughters? Too many times. Still, as soon as I heard about Dan, I called my boys to tell them the story. You have to remember this, I told them. This is what happens. Drinking and swimming don’t go together. Neither does the decision to suddenly scale a fence in the middle of the night, or jump into a pool with seven friends who are also intoxicated.
How parents deal with these tragic stories and talk about them with their own kids is critical. It shouldn’t be, “Oh, wow, I’m so glad that wasn’t my child.” Or, “My teenager would never have done that.” Because you don’t know. Instead, you have to be proactive. You have to stuff their minds with real stories, real consequences, and then you have to do it again—over dinner, after soccer practice, before music lessons, and, yes, even when they complain they’ve heard it all before. You have to remind them: These things can happen anytime, and there are many different situations that can get them into trouble and that can end badly.
One of the reasons that repetition is so important lies in your teenager’s brain development. One of the frontal lobes’ executive functions includes something called prospective memory, which is the ability to hold in your mind the intention to perform a certain action at a future time—for instance, remembering to return a phone call when you get home from work. Researchers have found not only that prospective memory is very much associated with the frontal lobes but also that it continues to develop and become more efficient specifically between the ages of six and ten, and then again in the twenties. Between the ages of ten and fourteen, however, studies reveal no significant improvement. It’s as if that part of the brain—the ability to remember to do something—is simply not keeping up with the rest of a teenager’s growth and development.
The parietal lobes, located just behind the frontal lobes, contain association areas and are crucial to being able to switch between tasks, something that also matures late in the adolescent brain. Switching between tasks is nearly a constant need in today’s world of information overload, especially when you consider the fact that multitasking—doing two cognitively complex things at the same time—is actually a myth. Chewing gum and doing virtually anything else is not multitasking because chewing gum involves no real cognitive focus. Both talking on a cell phone and driving, however, do involve cognitive focus. Because there are limits to how many things the human brain can focus on at any one time, when someone is engaged in multiple cognitively significant activities, like talking and driving, the brain must constantly switch back and forth between the two tasks. And when it does, neither of those tasks is being accomplished particularly well.
The parietal lobes help the frontal lobes to focus, but there are limits. The human brain is so good at this juggling that it seems as though we are doing two tasks at the same time, but really we’re not. Scientists at the Swedish medical university Karolinska Institutet measured those limits in 2009 when they used fMRI images of people multitasking to model what happens in the brain when we try to do more than one thing at a time. They found that a person’s working memory is capable of retaining only between two and seven different images at any one time; this means that focusing on more than one complex task is virtually impossible. Focusing chiefly happens in the parietal lobes, which dampen extraneous activity to allow the brain to concentrate on one thing and then another.
The problem of having immature parietal lobes was illustrated in a segment on Good Morning America in May 2008 by the ABC TV correspondent David Kerley and his teenage daughter Devan. Using a course set up by Allstate Insurance, and with her father in the passenger seat, Devan, who had been driving for a year, was instructed about speed, braking, and turning and allowed to take a practice run through the course. Then she was given a series of three “distractions” to handle while navigating the course’s twists and turns. First, she was handed a BlackBerry and told to read the text on the screen while driving. She hit several cones. Next, three of her friends were put in the backseat and a lively conversation ensued. Devan hit more cones. Finally, Devan was handed a package of cookies and a bottle of water, and just passing the cookies around and holding the bottle of water caused her to run over several more cones. Multitasking is not only a myth but a dangerous one, especially when it comes to the teenage brain.
“Multitasking” has become a household word. The research in Sweden suggests that there are limits. Teenagers and young adults pride themselves on their ability to multitask. Have today’s teens and young adults imprinted on a multitasking world? Maybe. In studying how young adults these days handle distractions, researchers at the University of Minnesota have shown that the ability to successfully switch attention among multiple tasks is still developing through the teenage years. So it may not come as a surprise to learn that of the nearly six thousand adolescents who die every year in automobile accidents, 87 percent die because of distracted driving.
The question of whether today’s teens and young adults have a special skill set for learning while distracted was more formally tested in 2006 by researchers at the University of Missouri. They took twenty-eight undergraduates, including kids in their late teens, and asked them to memorize lists of words and then recall these words at a later time. To test whether distraction affected their ability to memorize, the researchers asked the students to perform a concurrent task—placing a series of letters in order based on their color by pressing the keys on a computer keyboard. This task was given under two conditions: when the students were memorizing the lists of words and when the students were recalling those lists for the researchers. The Missouri scientists discovered that simultaneous tasks affected both encoding (memorizing) and retrieving (recalling). When the keyboard task was given while the students were trying to recall the previously memorized words (which is akin to taking a test or exam), there was a 9 to 26 percent decline in their ability to memorize the words. The decline was even more if the concurrent distracting task occurred while they were memorizing, in which case their performance decreased by a whopping 46 to 59 percent.
These results certainly have implications for the teen bedroom during a homework night! I not-so-fondly remember walking in on my sons during evening homework time to find them with the television on, headphones attached to iPods, all the while messaging someone on the lower corner of their computer screens and texting someone else on their iPhones. It wasn’t a problem, they protested, when I suggested they concentrate on their homework, assuring me their course reviews for the next day’s exams were totally unaffected by the thirty-two other things they were doing at the same time. I didn’t buy it. So I buttressed my argument with the Missouri data. I put Figure 5 in this book in case you want to use it to make the same point to your teen.
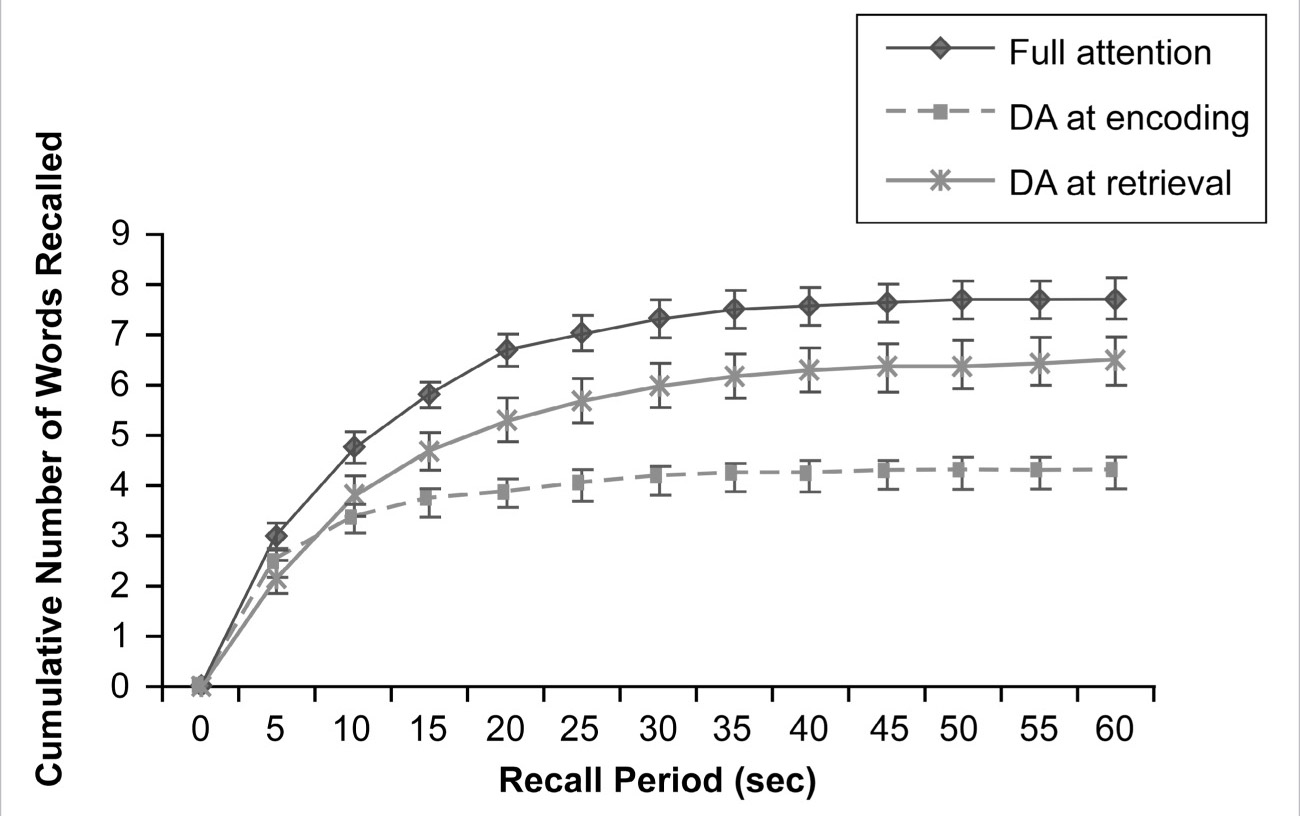
FIGURE 5. Multitasking Is Still Not Perfect in the Teen Brain: College students were tested under three conditions: No Distraction (full attention), Distracted Attention (DA) when memorizing (DA at encoding), and Distracted Attention when recalling (DA at retrieval). Students performed poorly when multitasking during recall, and even worse when they multitasked while memorizing.
Attention is only one way we can assess how the brain is working. There’s a lot more under the hood of the brain than just the four lobes, so returning to Figure 3 let’s start at the back, where we find the brainstem at the very bottom of the brain, attached to the spinal cord. The brainstem controls many of our most critical biological functions, like breathing, heart rate, blood pressure, and bladder and bowel movements. The brainstem is on “automatic”—you are not even aware of what it does, and you normally don’t voluntarily control what it does. The brainstem and spinal cord are connected to the higher parts of the brain through way station areas, like the thalamus, which sits right under the cortex. Information from all the senses flows through the thalamus to the cortex. Right below the cortex are structures called the basal ganglia, which play a big role in making coordinated and patterned movements. The basal ganglia are directly affected by Parkinson’s disease and account for the trembling and the appearance of being frozen, or unable to move, which are the hallmark symptoms of Parkinson’s patients.
As we move closer to the cortex, we encounter structures that together make up what is called the limbic system. The limbic system gets involved in memories and also emotions. A part of the brain we will talk about a lot in this book is the hippocampus. The hippocampus is a little seahorse-shaped structure underneath the temporal lobe. In fact the name “hippocampus” comes from the Latin word for “horse” because of the shape. The hippocampus is truly the brain’s “workhorse” for memory processing—it is used for encoding and retrieving memories.
So what do we know about our memory workhorse? It has the highest density of excitatory synapses in the brain. It is a virtual beehive of activity, and turns on with every experience. As we will explain later, the hippocampus in the adolescent brain is relatively “supercharged” compared with an adult’s.
The connection of the hippocampus to memory was recognized some six decades ago through the unforeseen consequences of one patient’s radical brain surgery. This surgery was performed in 1953 on a twenty-seven-year-old Connecticut man who, until his death several years ago, was known only by his initials, H.M. He underwent an experimental operation in an attempt to cure him of frequent and severe epileptic seizures. So incapacitating was H.M.’s epilepsy that he was unable to hold down even a factory job. When the Yale neurosurgeon William Beecher Scoville removed most of H.M.’s medial temporal lobe, which was causing his seizures, the operation appeared to be a success. By cutting away brain tissue in the area of the seizures, Scoville dramatically reduced their frequency and severity. In the process, though, he also removed a large portion of H.M.’s hippocampus. (That the hippocampus is critical for memory formation was unknown at the time; the case of H.M. shed much light on the subject.) What became clear when H.M. awoke was that while his seizures were by and large gone, so, too, was his ability to turn short-term memories into long-term memories. Essentially, H.M. could remember his past—everything before the time of the operation—but for the rest of his life he had no short-term memory and could not remember what happened to him, what he said or did or thought or felt or whom he met, in the decades following the surgery. H.M.’s loss, as often happens in the history of science, was neuroscience’s gain. For the first time researchers could point to a specific brain region (the temporal lobe) and brain structure (the hippocampus) as the seat of human memory.
Next door to the hippocampus, in another part of the limbic system under the temporal lobe, is another key brain structure, the amygdala, which is involved in sexual and emotional behavior. It is very susceptible to hormones, such as sex hormones and adrenaline. It is sort of the seat of anger, and when stimulated in animal experiments, it has been shown to produce rage-like behavior. The limbic system can be thought of as a kind of crossroads of the brain, where emotions and experiences are integrated.
A slightly unbridled and overexuberant immature amygdala is thought to contribute to adolescent explosiveness; this explains in part the hysteria that greets parents when they say no to whatever it is their adolescent thinks is a perfectly reasonable request. Cross that immature amygdala with a teen’s loosely connected frontal lobe, and you have a recipe for potential disaster. For example, the sixteen-year-old patient of a colleague of mine was so incensed when his parents said driving was a “privilege” (for which he did not yet qualify), and not a “right,” that he stole the car keys and drove away from the house. He didn’t get very far, though. He forgot the garage door was closed and plowed right through it. One of my colleagues also told me that, because he himself had three grown daughters, rather than sons, he had few “terrible teen tales” to tell. Then he reconsidered: “Oh, yes, there was the weekend we were away and the ‘couple of friends’ became a party that got out of hand, including the raid on our wine cellar, a minor fender bender with our stolen liquor in the trunk, and maybe a navel ring (which I never knew about until years later after it disappeared). But all’s well that ends well.”
3
Under the Microscope
If you pick out any random region of brain and look at it under a microscope, you’ll find it jam-packed with cells. In fact, there is almost no space between the billions of cells in the brain. Evolution made sure of that, putting to use every cubic micron wisely. A cell is the body’s smallest unitary building block, and each has its own command center, called a nucleus, a large oval body near the center of the cell. There are more than two hundred different types of cells making up every organ, tissue, muscle, etc. A unique cell type in the brain is the neuron. This is a cell we will talk about frequently in this book. Thoughts, feelings, movements, and moods are nothing more than neurons communicating by sending electrical messages to one another.
I remember my first time looking at brain cells under a microscope. In the mid- to late 1970s the only way to study changes in neurons, for instance the changes that occur during learning, was by looking through a microscope at individual cells over a given period of time. Today, we have amazing tools—brain imaging scans and specialized microscopes—that allow us to look into the brain and see cells and synapses change in real time. If you are learning something right now, as you read this, your neurons will change in about fifteen minutes, creating more synapses and receptors. Changes start within milliseconds of learning something new, and can take place over a period of minutes and hours. When I look at brain cells under a microscope, I think of the billions of neurons that are interconnected and how we’re still trying to figure out the wiring. What we know now is that no two human brains are wired exactly the same, and experience shapes us all differently. It’s the final frontier, our own internal frontier, and we’re just now beginning to see all the patterns.
There are 100 billion neurons in the human brain and you could place about 30,000 of them on the head of a pin, but placed end to end the neurons in just one person’s cortex would stretch for 100,000 miles—enough to circle the globe four times. At birth, we have more neurons than at any other time in our life. In fact, our brains are at their densest before birth, between the third and sixth months of gestation. Dramatic pruning of much of that gray matter occurs in the last trimester and first year of life. Still, by the time a baby is born, he or she has a brain brimming with neurons. Why? An infant’s overabundance of neural cells is needed to respond to the barrage of stimuli that comes with entering into the world. In response to all those new sights, sounds, smells, and sensations, neurons branch out in the baby’s brain, creating a thick forest of neural connections. So why aren’t all babies tiny Mozarts and Einsteins? Because when we are born, only a very small percentage of that overflow of neurons is wired together. The information is going in, being absorbed by the neurons, but it doesn’t know where to go next. Like someone plunked down in the middle of a strange and bustling metropolis, the infant brain is surrounded with possibilities and yet has no map, no compass, to navigate this strange new world. “All infants are born in a state of psychedelic splendor similar to an acid trip” is how Daniel Levitin, a neuroscientist at McGill University in Montreal, Canada, colorfully describes it.
A neuron responds to a stimulus with a burst of activity, called an action potential, which is actually an electrical signal that passes, in relay fashion, from the point of contact with the stimulus down the receiving limb of the neuron, called the dendrite, through the cell.
When we see the color red, smell a rose, move a muscle, or remember someone’s name, action potentials are happening.
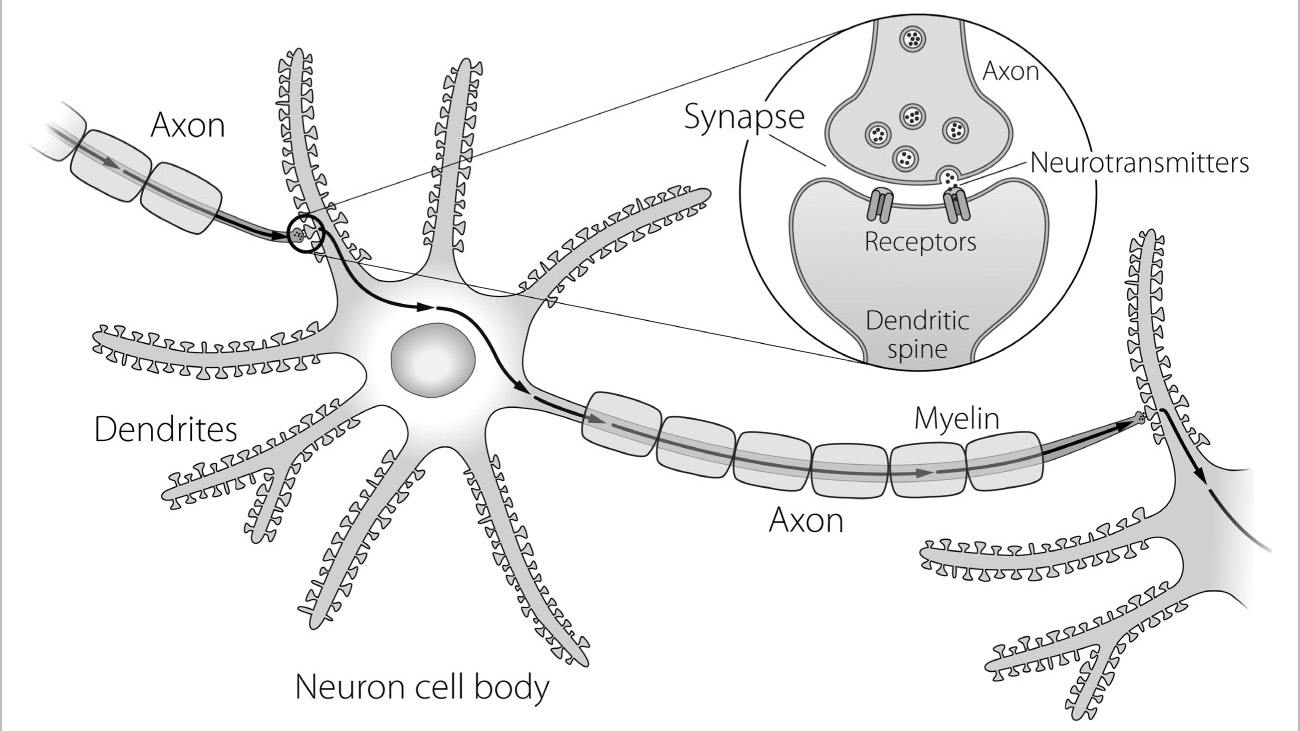
FIGURE 6. Anatomy of Neuron, Axon, Neurotransmitter, Synapse, Dendrite, and Myelin: Signals between cells flow in one direction, from an axon to a dendritic spine, through a synapse. Axons with myelin coating transmit signals faster than those without. At the synapse, a transfer of neurotransmitter molecules binds to the synaptic receptor on the spine.