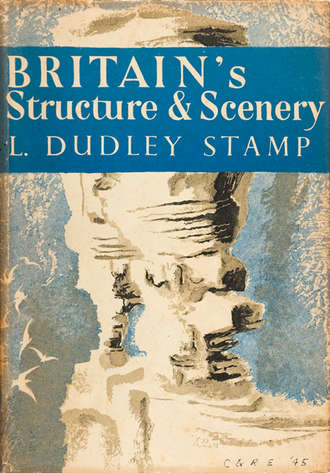
Полная версия
Britain’s Structure and Scenery
Presuming the original order to have been maintained, if the upper bed in one locality be traced laterally it may be found to pass under still higher beds in another so that the higher beds in the latter area are still newer. In this way a whole succession of strata may be built up—from the very oldest at the bottom to those still being formed at the top. Such a succession has, in fact, been constructed as a result of patient research and forms what is sometimes called the geological column. It must be realised that the rocks of the geological column are not to be found complete in any one area.
It must not be thought that if a hole were bored in the earth’s surface it would pass through all the rocks shown in the geological column. At the present day deposits of sand, silt and mud are being formed in the shallow waters near the estuaries or deltas of the great rivers of the world while other deposits, some of them consisting mainly of the hard parts of organisms living in the water, are being formed over the floors of most of the seas and oceans. In the lakes of the world other deposits are being laid down and even on parts of the land surface deep layers of sand and dust brought by wind are being spread over the older rocks. This is quite clearly seen where, as in the Culbin Sands of Morayshire shown on Plate XII, sand dunes are burying growing vegetation. These are all areas where deposition is taking place and where new strata are being formed. But over most of the land the rocks are being worn away by the combined action of rain, wind, sun, frost, running water and moving ice and its surface slowly but inevitably lowered. These are areas of denudation (Latin, denudo, I lay bare) and to them may be added the margins of the oceans where waves beat against the shores and wear them away. There will be no deposits in such areas to mark the present day and it was the same in the past. Thus beds present in one locality may be absent in another so that in the latter place there is a gap in the succession. Such a gap may indicate that the region concerned formed part of a land mass at the time in question or came otherwise under the influence of denudation. When the region again sank below sea-level and strata were again deposited it was perhaps after a lapse of many millions of years. Here is a “stratigraphical break” between the older and younger rocks. The younger rocks are said to rest “unconformably” on the older in those cases where the older had been folded and denuded in the meantime. A typical unconformity is shown in Plate IVB.
There is another difficulty in reconstructing the stratigraphical column. When one bed of rock or stratum is traced laterally it may change its character and unless the whole change can be traced and a limestone, for example, found to pass laterally into a shale or sandstone, it may be difficult to say that the limestone in the one place is of the same age as the sandstone in another. Of course if both types of rock contain the same fossils the answer is easy, but just as different habitats at the present day—muddy waters and clear lime-rich waters—may not have even a single species in common, so it happened in the past, and the fauna of a limestone may be completely different from the fauna of a bed of shale of the same age. To take a specific example, the beds known collectively as the Old Red Sandstone were laid down in freshwater lakes at the same time as the marine beds of the Devonian were being deposited elsewhere. In such cases the rare instances where the faunas are mixed, or there are “marine bands” representing incursions of the sea in the midst of a fresh-water succession, are invaluable in establishing the essential correlation. Thus the evidence which the geologist has to piece together is at the best fragmentary: it is rarely too that the rocks he wishes to study are “exposed” over large areas. In a country such as Britain the surface is hidden by soil and vegetation and only in some of the higher mountainous areas or along sea cliffs do the bare rocks outcrop at the surface. Elsewhere the geologist has to seek his evidence in quarries, mines, railway cuttings, well-borings, casual excavations for drains and sewers and even in some cases may be faced with the necessity of opening up a special pit in a crucial spot.
The rocks which are seen in the Stratigraphical Column were deposited over an immense period of time. Time is continuous, but there are certain natural phenomena which serve to divide it into definite units. The phenomenon of day and night serves to define one unit of time—the day ; the movement of the earth on its orbit round the sun defines another—the year. Larger units than the year are difficult to define but just as the astronomer uses a “light-year”—to define an enormous distance, so the geologist needs a larger unit than the year. The historian frequently takes the time between two important events to define a period ; thus when we talk of Tudor Times we mean the period when the Tudor kings were on the English throne, though we are able to define this period accurately in years—from the accession of Henry VII in 1485 to the death of Queen Elizabeth in 1603. The prehistorian is no longer able to measure his periods so accurately: he is obliged to define them in terms of the works of man in the periods concerned. The geologist, in his turn, has to deal with the vast periods of time which elapsed before the appearance of man on the surface of the earth ; for the definition or delimitation of such periods the year is an inadequate unit. No one would hand a traveller going on a long sea voyage a six-inch ruler and ask him to measure thereby the distances between the ports en route. Yet the voyager, by careful observation of time and direction, might be able to give a very fair account of the relative positions of the points touched, a close estimate of the distances between them and a good general account of their chief features. It would depend on his power of accurate observations and of using all the available evidence in its appropriate place. Thus the geologist has built up a good general picture of the evolution of the earth’s surface, a picture which is continually gaining in accuracy, and the geological time-scale is divided into a few great eras and a number of periods. The smallest unit of geological time is the hemera, usually named after a dominant animal or plant which was living at the time. A difficulty is that the animal or plant may have been local in its distribution, so that its absence from the sequence in a given locality is scarcely sufficient evidence that no deposition of beds was going on there at that time. A somewhat larger unit is the zone which, though named after a characteristic fossil, is usually to be defined by a characteristic associated series of fossils. A number of zones normally comprise a formation of rocks, while several formations make up a system of rocks. Thus we talk about the Chalk and the Lower Greensand as two of the formations in the Cretaceous System of rocks. But the word system refers to the rocks in the geological column: the rocks in a system were laid down in the period of time known as a geological period so that the measure of time concerned in this case is the Cretaceous Period. A number of periods are included in each of the four great eras into which geological time has been divided since the general appearance of life on the surface of the globe—i.e. since the deposition of the rocks which contain the earliest recognisable fossils. Even before that the earth had a long and complicated history which is gradually being unravelled and lowly forms of life doubtless existed but have left little or no trace.
On the general divisions of the geological time-scale and on the sequence of the periods all geologists are agreed though there is constant discussion regarding the exact definitions of the periods and whether a given bed of rock was laid down at the end of one period or the beginning of the next.
The layman is constantly demanding to be told the age of a given bed in years and amongst geologists themselves the age of the earth has always been a fascinating subject.
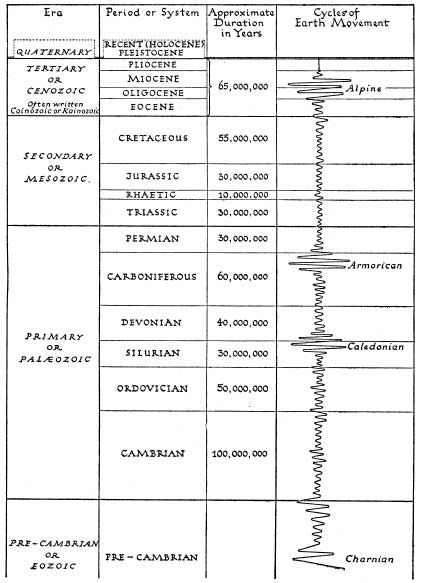
FIG. 4.—The Geological Column with the names of the geological periods and an approximate time scale in years.
One ingenious calculation worked out the amount of dissolved salts carried down to the sea every year by the rivers of the world and consequently how long it would have taken the ocean, presuming the water of the ocean to have been fresh originally, to have reached its present degree of salinity. A rough method at best, it breaks down as there is no evidence that the waters of the world ocean were originally fresh. In recent years a method of estimating geological time in years has been devised and used with considerable success. There are certain elements—the radioactive elements—which undergo disintegration at a constant but very slow rate which can be and has been measured. When a minute crystal of a radioactive mineral is enclosed in a larger crystal of certain other minerals—such as the dark mica, biotite—the emanations from the radioactive mineral cause a visible change in the surrounding mineral. When studied in section under the microscope the size of the zone of alteration, or “pleochroic halo,” affords a means of measurement of the time which has elapsed since the original formation of the rock. Another method is by the very accurate chemical analysis of the unaltered radioactive substance proportionate to the amount of the final end-products of its disintegration.
Piecing together the evidence, the geological column and the approximate duration of each period are in Fig. 4.
The names of the periods are reminders both of the richness of the British Isles in its varied geology where all the great systems are represented and also of the pioneer part played by British scientists in the geological field. The Cambrian takes its name from Cambria or Wales; the Ordovician and Silurian from two tribes of ancient Britons who lived on the Welsh borderland where these rocks are well developed and where they were first described. The name Devonian is from the county of Devon. Permian is a name which honours the pioneer studies of the British geologist Murchison in the province of Perm at the request of the Russians. Carboniferous (carbon- or coal-bearing) and Cretaceous (chalky) are descriptive of British conditions whilst the names of the divisions of the Tertiary are reminders of the Greek scholarship of Charles Lyell and his followers. The Jurassic (Jura Mountains) combines the older English Lias and Oolites; the Trias takes its name from the three-fold division typical of that system in Germany. Only the Rhaetic (Rhaetic Alps) is poorly represented in Britain. The Quaternary is not really comparable in duration or importance with the other great eras.
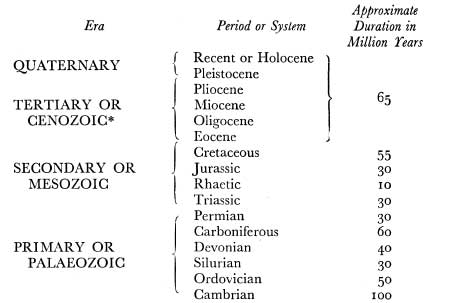
The coal-bearing strata of the Coal Measures form the upper division of the Carboniferous.
* Often written Cainozoic or Kainozoic.
CHAPTER 3
EARTH HISTORY—TIME AND LIFE
WE ARE fortunately living in one of the quiet periods of the earth’s history, though not at a time when it has been quiet for so long that the surface has been worn down to a dull and monotonous level. Although the accurate instrumental observation of earthquake phenomena demonstrates that the earth’s crust is rarely absolutely still, the occasional earthquakes which do occur, however severe they may be, are in reality but very gentle reminders of those periods in the history of the earth when the whole surface must have been torn by the most violent and long-continued cataclysms which bent and folded and broke the hardest rocks; which caused whole blocks of the earth’s crust to be thrust many scores of miles over other blocks; which caused continents to sink below the waves of the sea, or which caused vast tracts of the ocean bed to be raised to form the world’s highest mountains. The earthquakes of to-day are like the final murmurs of a great storm which has passed. Even they tend to occur in certain defined earthquake belts and are reminders that the earth’s crust has certain lines and zones of weakness whereas other parts are relatively stable.
If we exclude the great mountain-building movements which took place in the dim early days of the earth’s history—in the Pre-Cambrian—there have been three great periods of mountain building or “orogenesis” (Greek, oros mountain; genesis origin, creation) as far as Europe is concerned. Each of these has played a major part in determining the present-day features of Britain. These three great mountain-building periods were:
(a) at the end of the Silurian and beginning of the Devonian periods—the Caledonian earth-movements, so called because they built up the great mountains which have since been worn down to form the Highlands of Scotland (Caledonia);
(b) at the end of the Carboniferous and beginning of the Permian periods—the Armorican or Hercynian earth-movements, so called because they caused the great folding of the rocks seen in Brittany (Armorica) and the Hartz Mountains of Germany. In Britain they caused the uplift of the Pennines, the Malvern and Mendip Hills and folded the Coal Measures into basins.
(c) in the middle of the Tertiary era—the Alpine earth-movements, so called because they were responsible for the rise of the Alps as well as of many of the great mountain chains of the world to-day but which affected Britain much less than the previous movements.
Of the still earlier earth-movements at least one left very important marks in Britain—the Lewisian, which caused the folds in the rocks in the extreme north-west of Scotland and which may have been contemporary with the folding of the ancient rocks which peep from beneath a cover of later strata in the Charnwood Forest of Leicestershire.
In each case the mountain-building movements gathered strength slowly as with a developing storm and gradually reached a peak when the whole earth must have experienced a constant succession of gigantic earthquakes. Then gradually they must have died away again, the whole cycle stretching over an immense period of time. The result of these earth-building or orogenic movements was to form a series of gigantic wrinkles in the crust of the earth—these are the main mountain chains—between which are broad areas but little disturbed—the “tectonic” basins (Greek: tektonikos, related to building—i.e. not formed by later excavation. Sometimes these basins were below sea-level and became the areas of sedimentation in the succeeding periods, while the surrounding mountains as soon as formed were attacked by the forces of denudation which started to wear them down. So we get the idea of the geological history of the earth moving in great cycles. The first is what may be called the major cycle of denudation. This may be considered to begin when earth movements have caused land to rise above the level of the waters in the surrounding ocean. No sooner does this happen than the forces of sub-aerial denudation get to work. The heat of the sun heats the rocks and the different minerals of which they are composed have differential rates of expansion so that, especially with nightly cooling, the rocks are disrupted and a peeling or exfoliation (Latin: folium, a leaf) by successive layers takes place. This is sometimes called onion weathering and is well seen in hot dry countries at the present day. The direct action of the sun is called insolation.
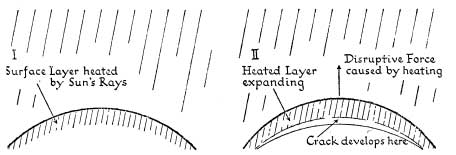
FIG. 5.—Diagrams showing the mechanism of exfoliation or onion weathering of rocks under the sun’s heat
Falling rain has a direct mechanical effect in washing away the finer particles, a less direct effect by dissolving some of the less stable minerals and an indirect effect by soaking into crevices. There it may be frozen and the water in changing to ice expands so that the crack is widened. This is the basis of frost action, through which great blocks may be split off from mountains and fall to lower levels as screes. Wind, too, plays its part by blowing away the finer dust and sand whilst strong wind armed with sharp sand particles is a powerful abrading agent. In newly formed mountain areas gravity itself plays a large part—for example in the formation of screes. Both in mountain areas and at lower levels landslides are by no means unknown. Gravity also causes the well-known phenomenon of soil creep, whereby soil gradually slides downhill. The process is seen at work in Plate 9B. Rain collects together to form mountain torrents which in turn unite to form swift rivers sweeping masses of debris always from higher to lower levels, from the land towards the sea. The eroding and transporting action of running water is paralleled in colder climates by the action of moving ice—glaciers which move slowly but inexorably down valleys or great icesheets which ride over the whole surface of the land, scooping out hollows where the rocks are soft, smoothing and polishing them where they are hard. In tundra lands the sub-soil remains permanently frozen whilst the surface thaws in summer and, where there are steep slopes, masses of sludge slide downhill, the whole process being called solifluction. On the margins of the seas and oceans wave action is a powerful force in wearing away the newly formed lands.
Whilst the major surface features of Britain owe their origin to the mountain-building movements of the past and to the character of the rocks which make up the land masses, many of the most striking scenic details are the result essentially of the different processes of weathering on varied rocks. In high mountain areas frost plays a large part and accounts for the angular rock surfaces such as those seen on Striding Edge (Plate XVIB) or in Snowdonia (Plate 8A) or on Cader Idris (Plate XXIX). Sometimes the sculpturing action of frost produces fantastic results, as in the well-known Sphinx Rock on Great Gable in Lakeland. Screes of fallen angular blocks and fragments of rock, most of them broken off by frost action, are a well-known feature in all mountain areas and sometimes dominate the landscape. Plate 30B shows the famous screes on the south side of Wastwater. Blocks of rock dislodged by the undercutting action of the sea and the action of rain form screes along many sea cliffs; a typical example from Cornwall has been shown in Plate 8B to illustrate the angle of rest assumed by loose rock of average character. The angle is much lower where rocks such as clay-shales become slippery when wet, and is lowest where the actual rock may “flow” when wet, which is the case with clay.
Onion weathering under the influence of the sun leaves hard, rounded cores of rock. In tropical countries, these may be almost true spheres; in this country such “cores” scattered over the country are familiar in many granite areas. A good example may be seen on Crousa Common (The Lizard, Cornwall), whilst the interesting weathering of granite, seen in such “tors” as those of Dartmoor (Plate XXVII) is to be ascribed mainly to the same action.
The most interesting results are seen where the original rock varies in hardness. A sandstone, for example, may be indurated along certain lines and the denuding agent whether wind, rain, running water or the sea finds out the pockets of softer sand and scoops them out. The interestingly fretted rock shown in Plate VI is actually the result of the action of the sea, but a very similar appearance might be due to wind action. Where a rock is fractured rain washes out the loose, crushed rock and produces striking cliffs such as those shown in Plate IB. Even in Lowland Britain the “High Rocks” of Tunbridge Wells are simple examples of differential weathering.
Immediately after a great earth-building movement the deposits which fill the hollows—the tectonic valleys and basins—are coarse and often consist of angular blocks which are actually screes and may become consolidated to form a “breccia.” Beds of roughly rounded boulders and large pebbles may be deposited by swift streams to become consolidated later as conglomerates and pebble beds. Plate VIIIB shows an example from the Lake District of such boulders being swept down by a stream in flood. As time goes on the mountains are worn down, yield less material and the beds laid down in the basins and seas become finer grained in character—sands and silts and muds, which may become consolidated respectively into sandstones, siltstones and shales. In the later stages of the cycle muds and clays will definitely predominate and when the lands have been worn down almost to plains (called “peneplanes” or “peneplains”—Latin: pene, almost) they will yield so little sediment that the waters of the surrounding seas may become quite clear. These conditions of clear tranquil water are those under which corals flourish and also other organisms which build up their hard parts of calcium carbonate; thus the deposits then formed are often limestones. The cycle of denudation on the land and of sedimentation in the water is brought to a close by earth movements, it may be slight at first, which herald the oncoming of a new storm. More often the major cycle of events is varied by minor earth movements—it may be the so-called “eustatic” movements, not of folding of the earth’s crust, but of the gentle elevation or depression of blocks of it relative to the level of the waters—so that minor cycles of sedimentation occur within the major. This is well illustrated in the geographical evolution of the British Isles.
So far nothing has been said regarding what is now known of the structure of the earth as a whole. It cannot be too forcibly stated that the old concept of a solid crust, rather like the skin of an apple, covering a molten interior, is entirely wrong and that the simple deduction that the whole was cooling and contracting so that wrinkles—which were the mountain ranges—were being formed just as when an apple dries is equally false. We now know that there is a central sphere, solid and very heavy and probably consisting of an alloy of iron and nickel—thus agreeing in composition with some of the meteorites which from time to time fall on the earth’s surface. This iron-nickel core accounts for the magnetic phenomena of the earth. Enveloping this is the crust, in all about 700 miles thick—a figure which may be compared with a height of 5 miles for the highest mountain and a depth of 6 miles for the deepest ocean. It is well known that there is a rapid increase in temperature as one goes downwards in the crust so that even in a deep mine it is almost unbearably hot.
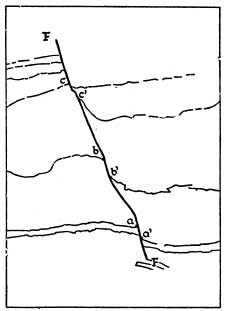
FIG. 6.—Diagram of the Fault shown in Plate IA. This is a typical example of a very small normal fault. The fault plane separates the downthrow side on the right from the upthrow side on the left. The angle which the fault plane makes with the vertical is the hade; the vertical displacement (here only a few inches, though in big faults it may be thousands of feet) is the throw. Normal faults occur under tension whereas thrust faults and structures such as are shown in Fig. 72 occur under extreme compression.
It does not necessarily follow that the solid core of the earth is extremely hot, since it is now known that heat accumulates in the lower layers of the crust through radioactivity. What is important is not the temperature of the central core but of the crust. At no great depth the temperature must be such that all rocks would be molten were they not kept in a solid or more probably a plastic condition by the pressure of the solid rocks above. Towards the end of a major cycle of denudation, however, so much material has been removed from one part of the surface of the crust to another that the pressure is lessened over the land. Some of the underlying heated layer becomes actually molten and seeks to find weak spots or lines in the crust through which it can escape. It may reach the surface and be poured out through the craters of volcanoes (volcanic eruptions) or through cracks in the surface (fissure eruptions) as lava. Some of the molten rock does not reach the surface but forces its way into cracks and there consolidates as wall-like masses or dykes; or it may force its way parallel to the bedding planes of sediments to form sills. A striking example of an old volcano with associated sill is found in Arthur’s Seat, Edinburgh, shown in Plate XVIA. In all these cases the molten rock bakes and hardens the rocks through which it passes—it changes their form by its contact (Greek: meta- change,