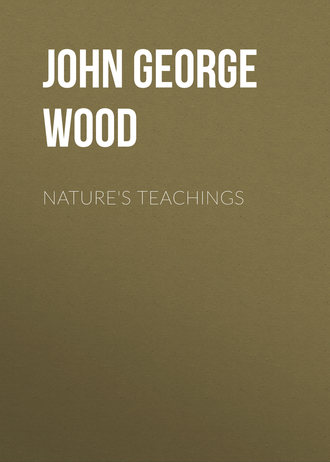
Полная версия
Nature's Teachings
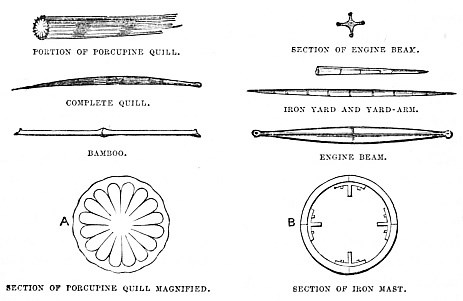
But were the quill merely a hollow tube filled with pith, it would be too weak to resist the strain to which it is often liable. Consequently it is strengthened by a number of internal ribs, composed of the same horny material as the outer coat, and arranged in exactly the same way as those of the mast.
There are yet other points in the structure of the Porcupine quill which might be imitated with advantage in the mast. In the first place, the internal ribs are much more numerous than those of the mast, but they are very much thinner, and taper away from the base, where the greatest strain exists, to the end, where they come to the finest imaginable edge. This modification of structure enables the outer shell of the quill to be exceedingly thin and light, and, moreover, gives to the whole quill an elasticity which is quite wonderful, considering its weight and strength.
Then, in the iron mast the exterior is quite smooth, whereas in the Porcupine quill it is regularly indented, exactly on the principle of the corrugated iron, which combines great strength with great lightness. And I cannot but think that our iron masts might be made both lighter and stronger if the shell were thinner, the internal ribs made like those of the Porcupine quill, and the shell corrugated instead of being quite smooth. The internal cells of the quill are, of course, not needed in the mast, as they are intended for nutrition, and not for strength.
Being on this subject, we may take the shape of the Porcupine quill, and compare it with that of the ship’s yard. It will be seen that the two are so exactly similar in form that the outline of one would answer perfectly well for the other. The only perceptible difference is, that in the ship’s yard both ends are alike, whereas in the Porcupine quill the end which is inserted in the skin is rounded and slightly bent, while the other end is sharply pointed.
The principal point to be noticed in the form of both quill and yard is, that they become thicker in the centre, that being the spot on which the greatest strain comes, and which, in consequence, needs to be stronger than any other part. While holding and balancing the pole which Blondin uses to preserve his balance when walking on the high rope, I was struck with the fact that the pole, which is heavily weighted at each end, had to be strengthened in the middle, exactly on the principle of the Porcupine quill and the ship’s yard. It could not, of course, be thickened, as the hands could not grasp it, but it had to be furnished with additional strengthening. And the necessity of such strengthening is evident from the fact that on one occasion the pole did break in the middle, so that any one of less nerve and presence of mind must have been killed.
Bearing in mind, then, that in a rod or pole the centre is the part which most requires to be strengthened, we can see, in cases too numerous to mention, how art has followed, though perhaps unconsciously, in the footsteps of nature. Take, for example, the beam of a steam-engine, such as is given in the sketch, and for which the great engine at Chatham acted as model. The reader will observe that in this case the beam is gradually thickened towards the centre, the ends, where the strain is slightest, being comparatively small.
Another point also must be noticed. Equal strength could have been obtained had the beam been solid, but at the expense of weight, and consequent waste of power. Lightness is therefore combined with strength by making the beam consist of a comparatively slight centre, but having four bold ridges, as shown in the section given in the accompanying illustration. This plan, as the reader will see, is exactly the same as that which is adopted in the iron mast and porcupine quill, except that the ridges are external instead of internal. The same mode of construction is employed in ordinary cranes, the principal beam of which is almost identical in form with that of the engine, both being thickest in the centre, and both strengthened with external ridges.
There are also other analogies between the hollow mast and natural objects. Keeping still to the animal world, we find the quill feathers of the flying birds to supply examples of the combination of great strength with great lightness and very little expenditure of material. Their wing bones, too, are hollow, communicating with the lungs, and are consequently light as well as strong.
Passing to the vegetable world, we find a familiar example of this structure in the common Wheat Straw. The ripe ear is so heavy, when compared with the amount of material which can be spared to carry it, that if the stalk were solid it would give way under the mere weight of the ear. Moreover, the full-grown corn has to endure much additional weight when wetted with rain, and to resist much additional force when bowed by the wind, so that a slight and solid stalk would be quite inadequate to the task of supporting the ear.
The material of the stalk is therefore utilised in a different manner, being formed into a hollow cylinder, the exterior of which is coated with a very thin shell of flint, or “silex” as it is scientifically termed. The result of this structure is that the stem possesses strength, lightness, and elasticity, so as to be equal to the burden which is laid upon it.
Then there is the common Bamboo, which is little more than a magnified straw, being constructed in much the same manner, and possessing almost the same constituents of vegetable matter and silex.
Perhaps the most extraordinary of the tubal system is to be found in the remarkable plant of Guiana called by the natives Ourah, and scientifically known by the name of Arundinaria Schomburgkii. Like the bamboo, it grows in clusters, and has a feathery top, which waves about in the breeze. But, instead of decreasing gradually in size from the base upwards, the Ourah, although it runs to some fifty feet in height, is nowhere more than half an inch in diameter. The first joint is about sixteen feet in length, and uniform in diameter throughout.
It is scarcely thicker than ordinary pasteboard, and yet so strong and elastic is it, that it can sustain with ease the weight and strain of its feathery top as it blows about in the breeze. The natives of certain parts of Guiana use this reed as a blow-gun, and I have a specimen, presented to me by the late Mr. Waterton, which is eleven feet in length.
So the reader will see that when engineers found that hollow iron beams were not only lighter, but stronger than solid beams, they were simply copying the hollow beams formed by Nature thousands of years ago.
Another great improvement in ship-building now comes before us.
We have already seen that the earliest boats were merely hollowed logs, just as Robinson Crusoe is represented to have made. But these had many disadvantages. They were always too heavy. They were liable to split, on account of flaws in the wood, and if a large vessel were needed, it was difficult to find a tree sufficiently large, or to get it down to the water when finished.
So the next idea was to build a skeleton, so to speak, of light wooden beams, and to surround it with an outer clothing, or skin, if it may be so termed. As far as I know, the two original types of this structure are the Coracle of the ancient Briton, and the birch-bark Canoe of the North American Indian, and it is not a little remarkable that both exist to the present day, with scarcely any modification.
The Coracle has been already represented on page 22. It is, perhaps, or was in its original form, the simplest boat in existence, next to the “dug-out.” In the times of the very ancient Britons, who were content with blue paint by way of dress, and lived by hunting and fishing, the Coracle was a basin-shaped basket of wicker-work, rather longer than wide, and covered with the skin of a wild ox. This was sufficiently light to be carried by one man, and sufficiently buoyant to bear him down rapids, if he were a skilful paddler, and, of course, formed a considerable step in civilisation.
The modern Coracle is identical in form, and almost in material. The frame is still oval and basin-shaped, and made of wicker, but the outer covering is not the same. An ox-hide is an expensive article in these days, and, especially when wetted, is very heavy. So the modern Coracle builder covers the wicker skin with a piece of tarpaulin, which is much cheaper than the ox-hide, much lighter, is equally water-tight, and has the great advantage of not absorbing moisture, so that it is as light after use as before.
The Esquimaux make a boat on very similar principles. It is simply hideous in form, resembling a huge washing tub in shape, but, as it is only intended for the inferior beings called women, this does not signify.
Best, most perfect, and most graceful of all such boats is the Birch-bark Canoe of the North American Indians, whose shape has evidently been borrowed from that of a fish. I have seen many of these canoes, and have now before me several models which are exactly like the originals, except in point of size. Instead of being mere elongated bowls, like the coracle, they are long and slender, swelling out considerably in the middle, and coming to an almost knife-like edge at each end. Both stem and stern are alike, so that the canoe can be paddled in either direction, and, as one of the paddlers always acts as steersman, no rudder is needed.
The mode of construction is perfectly simple. The labour is divided between the sexes: the women cut large sheets of bark from the birch-trees, scrape and smooth them, and then sew them together, so as to form the outer skin, or “cloak” as it is called, of the canoe. Meanwhile the men are making the skeleton of strips of white cedar-wood, and binding them into shape with thongs made of the inner bark of the same tree, just like the “bass” of our gardeners. The “cloak” is then gradually worked over the skeleton, sewn into its place, and the canoe is finished. A figure of this canoe, as completed, is given in the same illustration as that which represents various forms of boat, page 7.
The last improvement is that which was caused by the necessity for large vessels, when planks or iron plates were fastened over the skeleton. But, in all these cases, the vessel is built on the principle of the thorax of a vertebrate animal, that of the whale or a fish being an admirable example. It only needs to take the skeleton of a whale, turn it on its back, and the ribs will be seen to form an almost exact reproduction of those of any ship being built in the nearest dockyard.
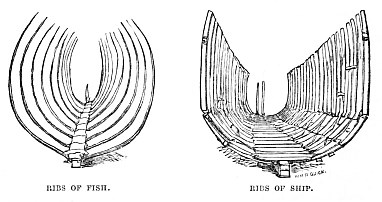
I have now before me the spine and ribs of a herring. The fish was over-boiled, and the flesh fell off the bones as it was being lifted out of the dish, leaving most of the ribs in their places. When held with the spine downwards, and viewed from one end, the resemblance to the framework of a ship is absolutely startling, the ribs representing the beams, and the spine taking the place of the keel. I have also before me a sketch representing a section of a Fijian canoe, and it is remarkable that even the very curve of the ribs of the herring is reproduced in those of the canoe.
Whether the Fijians derived this peculiar and beautiful curve from the ribs of a fish I cannot say, but think it very likely.
A still greater improvement in ship-building now comes before us, and this also has been anticipated both in the animal and vegetable kingdoms. There are so many examples of this anticipation that I can only give one or two.
The improvement to which I refer is that which is now almost universally employed in the construction of iron ships, namely, the making the outer shell double instead of single, and dividing it into a number of separate compartments. Putting aside the advantage that if the vessel were stove, only one compartment would fill, we have the fact that the ship is at the same time enormously strengthened and very light in proportion to her bulk.
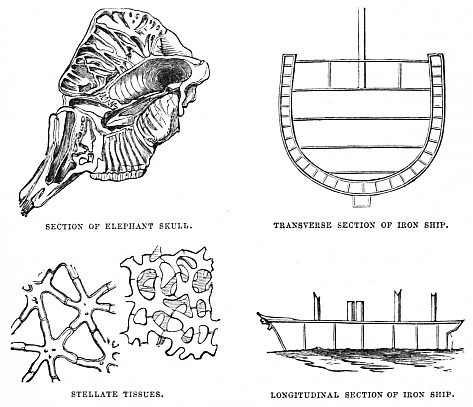
Perhaps the best, and certainly the most obvious, example of this principle in the animal world is to be found in the skull of the Elephant. The enormous tusks, with their powerful leverage, the massive teeth, and the large and weighty proboscis, require a corresponding supply of muscles, and consequently a large surface of bone for the attachments of these muscles. Now, were the skull solid in proportion to its requisite size, its weight would be too much for the neck to endure, however short and sturdy it might be. The mode of attaining expanse of surface, together with lightness of structure, is singularly beautiful.
Perhaps some of my readers may not be aware that the bone of the skull consists of an outer and inner plate, with a variable arrangement of cells between them. In many animals, such, for example, as man, where the jaws are comparatively feeble, and the teeth small and light, the size of the skull is practically that of the brain, to which it affords a covering. The same structure may be observed in the skull of the common sparrow, where, as in man, the two bony plates are set almost in contact.
But in the elephant these external and internal plates are set widely apart, and the space between them is filled with bony cells, much resembling those of a honeycomb. They are, in fact, just the same cells as those which exist in the skull of man and sparrow, but they are very much enlarged, and in consequence give a large surface, accompanied with united strength and lightness.
There are many other examples in the animal kingdom, but our limited space will not allow them to be even mentioned.
As to the vegetable examples of this principle, they are so multitudinous that only a very slight description can be given of them.
I suppose that most boys have seen a “cane” (whether they have felt it or not is not to the purpose), and some boys have made sham cigars from pieces of cane. In either case they must have noticed that the cane is not solid, but is pierced with a vast number of holes, passing longitudinally through it, and is, in fact, a collection of little tubes connected and bound together by a common envelope.
The Sugar-cane, if cut across, is seen also to consist of multitudinous cells, which, however, are not hollow, but filled with the sweet liquid from which sugar is obtained by boiling. Then there are many of our common English plants, like the ordinary rush or reed, which are very slight in diameter in comparison with their length, and in which the cells are still further strengthened and lightened by the projection of their sides into a number of points which meet each other, and leave interstices between them. This modification of the cellular system is called “Stellate” (or star-like) Tissue, and two examples of it are given in the illustration, one being taken from the common rush, and the other from the seed-coat of the privet. A very good specimen of stellate tissue may be obtained by cutting a thin section of the white inner peel of the orange.
CHAPTER IV.
SUBSIDIARY APPLIANCES.—Part II
The Cable and its Variations.—Material of Cables.—Hempen and Iron Cables, and Elasticity of the latter.—Natural Cables.—The “Byssus” of the Pinna and the common Mussel.—The Water-snail and its Cable.—A similar Cable produced by the common White Slug.—The Principle of Elasticity.—Elastic Cable of the Garden Spider.—Tendrilous Cables of the Pea and the Bryony.—The Vallisneria, and its Development through the Elastic Cable.—Proposed Submarine Telegraph Cable.—The Anchor, Grapnel, and their Varieties.—Natural Anchors.—Spicule of Synapta.—The Grapnel, natural and artificial.—Ice-anchor and Walrus Tusks.—The Mushroom Kedge.—The Flesh-hook.—Eagle-claw.—The Grapple-plant of South Africa.—The Drag.
AMONG the most important accessories to a ship are the Cable, by which she can be anchored to the bed of the sea, and the ropes called “warps,” by which she can be fastened to the land.
Perhaps my readers may not know the old riddle—“How many ropes are there on board a man-of-war?” The non-nautical individual cannot answer, but the initiated replies that there are only three, namely, the man-rope, the tiller-rope, and the rope’s-end, all the others being “tacks,” “sheets,” “haulyards,” “stays,” “braces,” &c.
Formerly cables were always made of hemp, enormously thick, and most carefully twisted by hand. Now, even in small vessels, the hempen cable has been superseded by the iron chain, and this for several reasons.
In the first place, it is much smaller in bulk, and therefore does not occupy so much room. In the next place, it is even lighter than the hempen cable of corresponding strength; and, in the third, its specific gravity—i.e. its weight when compared with an equal bulk of water—is so great, that when submerged, it falls into a sort of arch-like form, and so attains an elasticity which takes off much of the strain on the anchor, and protects it from dragging.
We will now look to Nature for Cables.
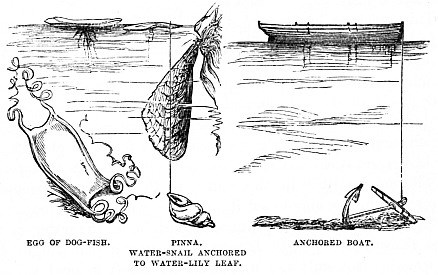
The natural cable which will first suggest itself is evidently that of the Pinna Shell (Pinna pectinata), which fixes its shell to some rock or stone with a number of silk-like threads, spun by itself, and protruding from the base, just as a vessel on a lee shore throws out a number of cables. The threads which compose the “byssus,” as it is called, are only a few inches in length, and apparently slight. They are, however, really strong, and by acting in unison enable the shell, though sometimes two feet in length, to be held firmly to the rock. I may here mention that they have been occasionally woven into gloves, and other articles of apparel, to which their natural soft grey-brown hue gives a very pleasing appearance.
A still more familiar instance of a natural marine cable is given by the common Mussel, which can be found in thousands on almost every solid substance which affords it a hold. Even copper-bottomed ships are often covered with Mussels, all clinging by their natural cables, and it is thought that the cases which sometimes occur of being poisoned by eating Mussels, or “musselled,” as the malady is called by the seafaring population, are due to the fact that the Mussels have anchored themselves to copper, and have in consequence imbibed the verdigris.
Passing from salt to fresh water, we come to a natural cable which is very common, and yet, on account of its practical invisibility, is almost unknown, except by naturalists. I refer to the curious cable which is constructed by the common Water-snail (Limnæa stagnalis), which has already been mentioned in its capacity of a boat.
This creature has a way of attaching itself to some fixed object, such as a water-lily leaf, by means of a gelatinous thread, which it can elongate at pleasure, and by means of which it can retain its position in a stream, or in still water can sink itself to the bottom, and ascend to the same spot. This cable seems to be made of the same glairy secretion as that which surrounds the egg-masses which are found so plentifully on leaves and stones in our fresh waters, and, like that substance, is all but invisible in the water, so that an inexperienced eye would not be able to see it, even if it were pointed out.
Slight, gelatinous, and almost invisible in the water as is this thread, its strength is very much greater than might be supposed. Not only can a mollusc be safely moored in the water by such a cable, but it can be actually suspended in the air, as may be seen from a letter in Hardwicke’s Science Gossip for 1875, p. 190:—
“Last summer (September 29) I met with the following unusual fact. In a green-house, from a vine-leaf which was within a few inches of the glass … a slug was hanging by a thread, which was more than four feet in length, not unlike a spider-web, but evidently much stronger.
“The slug was descending by means of this thread, and, as the glutinous matter from the under part of the body was drawn out by the weight of the creature, it was consolidated into a compact thread by the slug twisting itself in the direction of the hands of a clock, the power of twisting being given by the head, and the part of the body nearest the head being turned in the direction of the twist. There was no tendency to turn in the contrary direction. Evidently the thread became hard as soon as it was drawn away from the body.
“By wetting the sides of slips of glass, I secured two specimens of the thread. In one of these, part was stretched, and part quite loose, the latter appearing flat when seen through a microscope. The thread, which was highly elastic, was increased about three inches in a minute. The slug was white, and about an inch and a half in length.”
Now we come to the elastic system of the Chain Cable, and find it anticipated in Nature in various ways.
One curious example was that of a Spider, which found its wheel-like net in danger from a tempestuous wind. The Spider descended to the ground, a depth of about seven feet, and, instead of attaching its thread to a stone or plant, fastened it to a piece of loose stick, hauled it up a few feet clear of the ground, and then went back to its web. The piece of stick thus left suspended acted in a most admirable manner, giving strength and support, and at the same time yielding partly to the wind.
By accident the thread became broken, and the stick, which was about as thick as an ordinary pencil, and not quite three inches in length, fell to the ground. The Spider immediately descended, attached another thread, and hauled it up as before. In a day or two, when the tempestuous weather had ceased, the Spider voluntarily cut the thread, and allowed the then useless stick to drop.
A curious example of the elastic cable is seen in the egg-case of the Dog-fish, which is given on page 35. The egg-case is formed like that of the common skate, and has a projection from each of its angles. But the projections, instead of being mere flattened horns, are lengthened into long elastic strings, tapering towards the ends, and twisted spirally, like the tendrils of a grape-vine.
These tendril-like appendages twist themselves round seaweeds and other objects, and, on account of their spiral form, can hardly ever be torn from their attachments. Sometimes after a storm the egg is thrown on the shore, still clinging to the seaweed, but to find an egg detached is very rarely done.
I have already mentioned the tendrils of the vine, and their great strength. The reader may remember the corresponding cases of the Pea and the Bryony, the latter being a most remarkable example of the strength gained by the spiral form. It clambers about hedges, is exposed to the fiercest winds, has large and broad leaves, and yet such a thing as a Bryony being blown off a hedge is scarcely, if ever, seen. I never saw an example myself, though I have had long experience in hedges.
Another excellent example of this principle is found in the Vallisneria plant, which of late years has become tolerably familiar to us through the means of fresh-water aquaria, though it is not indigenous to this country.
In this plant the elastic power of the spiral cable is beautifully developed. It is an aquatic plant, mostly found in running waters, and has a most singular mode of development. It is diœcious—i.e. the male, or stamen-bearing, and the female, or pistil-bearing flowers, grow upon separate plants.
It has to deposit its seeds in the bed of the stream, and yet it is necessary that both sets of flowers should be exposed to the air and sun before they become able to perform their several duties. Add to this the fact that the male flower is quite as small in proportion to the female as is the case with the lac and scale insects, and the problem of their reaching each other becomes apparently intricate, though it is solved in a beautifully simple manner.
Fertilisation cannot be conducted by means of insects, as is the case with so many diœcious terrestrial plants, and it is absolutely necessary that actual contact should take place between them. This difficult process is effected as follows:—