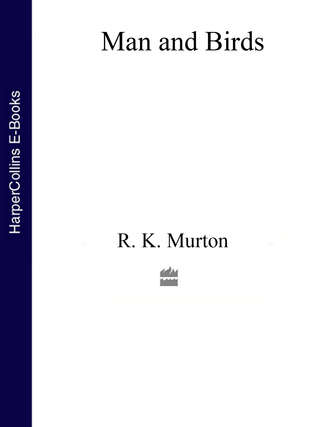
Полная версия
Collins New Naturalist Library
Fig. 4 shows the autumn population, in decreasing order of size, of the grey partridge on the Norfolk estate against the percentage of birds dying in winter, either as a result of shooting or through natural causes. Similar data for the red-leg are also detailed, these being plotted against the appropriate years for grey and not in their own size order. The figure demonstrates in the case of the grey partridge that mortality due to shooting and other factors combined is positively correlated with the size of the autumn population, that is, it is density-dependent. The percentage of birds shot is even more strongly correlated with the numbers available for shooting and is also correlated with the total mortality. In contrast, natural mortality is not correlated with autumn numbers nor with shooting loss. That death by shooting should be adjusted to the autumn population is to be expected, since the people involved determine their activities according to the prospects; in years when autumn numbers are small, with a low young to old ratio, shooting is voluntarily abolished or curtailed. This attitude is mistaken for the simple reason that, in spite of shooting, additional animals have in any case to be lost to ensure stability in the spring, and if none are shot more disappear through other causes. This is well shown in Fig. 4 for the year 1954 when little or no shooting occurred and the level of natural mortality was raised. As a result the death-rate was at virtually the same level as it was in 1952 and 1955, when extensive shooting took place. Although enough animals are shot to ensure a density-dependent controlling effect on the winter population, this is only so because shooting obscures other causes of death. Because shooting may actually account for deaths it is not the reason why these occur: they would also occur in the absence of shooting. Jenkins reached this same conclusion for partridges he studied on Lord Rank’s estate at Micheldever in Hampshire, and he and his colleagues (Jenkins, Watson and Miller) have more recently found that the same applies in the case of the grouse – the details are shown graphically in Fig. 5. The practical conclusion to be drawn from these studies is that people could as a rule enjoy more intensive shooting without detriment to game stocks – though this conclusion caused scepticism among many shooting men. It is ironical, therefore, that my colleagues and I found exactly the same principle to apply to a pest bird, the wood-pigeon. The number of these shot during organised battues in February was always less than the number which had to be lost to bring about stability in relation to clover stocks. We concluded that winter shooting was not controlling the population, nor in the circumstances did it reduce crop damage. Again this conclusion caused scepticism among many shooting men who could not appreciate that causes of death are compensatory, not additive. Only if more birds are shot than will be lost in any case can shooting become a controlling factor, in the sense that it will reduce numbers to lower levels in the next season.
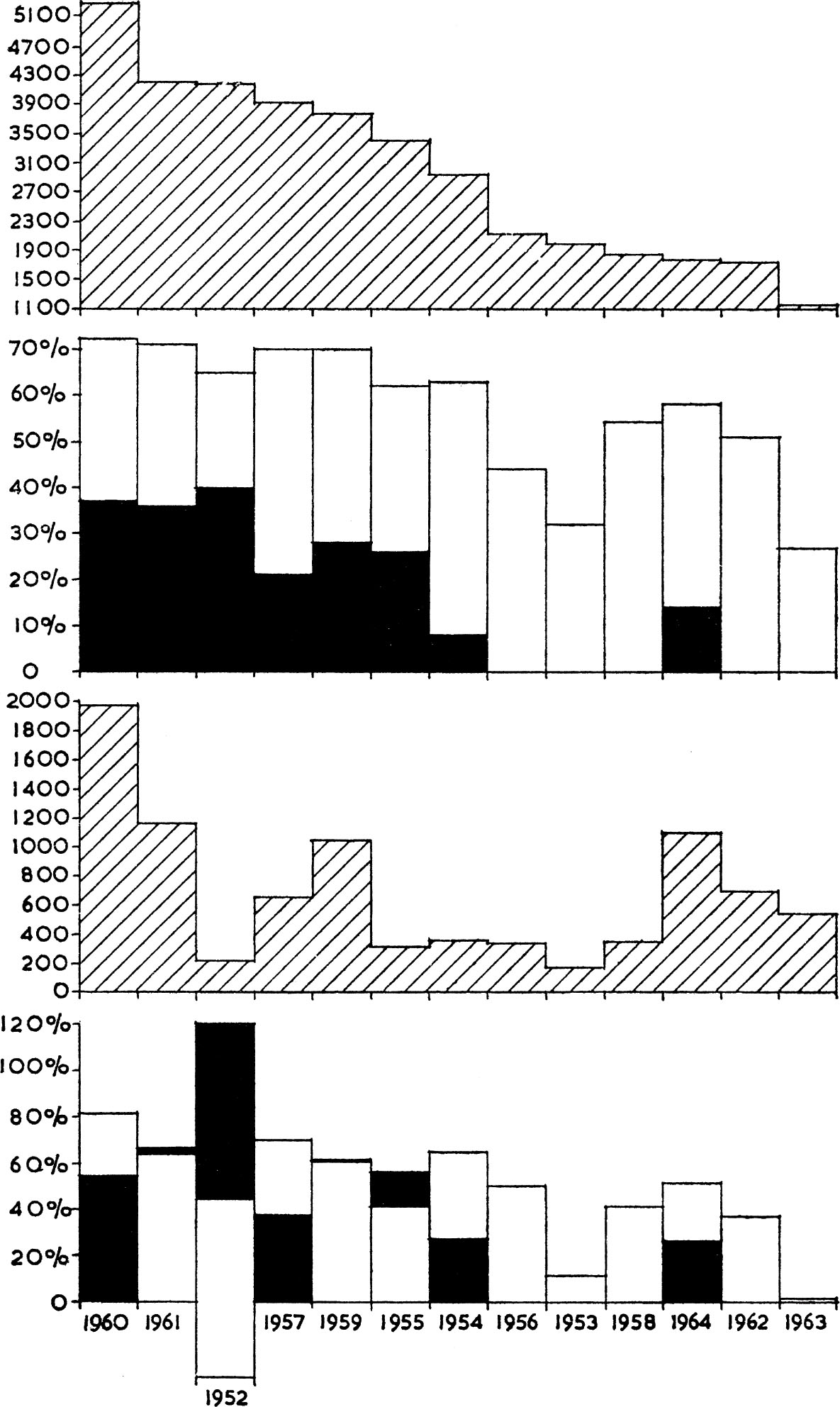
FIG. 4. Relationship between the autumn population of the grey partridge (top hatched histogram) and red-legged partridge (third histogram from top, hatched) with the percentage of these populations lost between autumn and the following spring represented in the histograms below each. The percentage of birds lost is given by the open columns, and the percentage of these birds which were shot by the solid black. Loss due to other causes, is therefore, the difference between black and open parts of the columns.
[For the grey partridge the total mortality in winter is correlated with the autumn population being heavier in years of higher autumn numbers r11 =0.827. The percentage shot each winter is correlated with the autumn population r11 =0.909. The percentage shot is not correlated with the number lost for other reasons r11 = —0.420.
For the red-legged partridge the total mortality in winter is less correlated with the autumn population of red-legs, r11 =0.583, and more correlated with the autumn population of grey birds r11 =0.747. The number of red-legs shot bears no relation to the autumn population of red-legs but is related to the autumn population of the grey, r11 =0.789.]
The reason for some of the apparently anomalous results with the red-leg in which a higher proportion of birds was sometimes shot than was present in autumn is that the birds were moving into the area. Thus in 1952 the autumn population was 214, 259 birds were shot yet the spring population was 120, i.e. 121% of the autumn population was shot, there was a 77% loss not due to shooting (theoretically the difference between the total loss from autumn to spring=44%; with the loss due to shooting subtracted = — 77%, and this has to be shown by drawing the column below the base line. (Data derived from Table 1, from Middleton & Huband 1966).
The number of red-legged partridges shot on the Norfolk estate has borne only a slight correlation with the autumn numbers (Fig. 4). As the red-leg was much rarer than the grey, any decision on the numbers to be shot was made relative to the latter species, or more accurately to the total numbers of both species, rather than to the autumn population of the red-leg. For this reason the percentage of red-legs shot was fairly strongly correlated with autumn numbers of grey birds, while the total winter mortality (shooting plus natural) was slightly less strongly correlated. This illustrates how the amount of predation (in this case shooting) suffered by a species may be determined by the availability of similar prey. In such circumstances, the situation may arise where undesirably large numbers are lost by accident, even resulting in the extinction of a species.
Still considering Fig. 4, it is possible to imagine that this represented a species where the shooting had been done for pest control and not for sport, and that it was supported by a bounty. It becomes evident that a bonus scheme, unless it actually results in more animals being killed than would die in any case, would in this case prove a complete waste of money as a means of controlling numbers. It could only be justified if the animals concerned were killed before they caused damage. While the undesirability of a direct subsidy is fairly evident, there are often cases where grant-aid is paid in an indirect manner which obscures its futility. Variations in kill dependent on population size, and hence variations in subsidy, would be anticipated in different seasons – yet it usually happens that the amount claimed for a subsidy stays fairly constant. This is so in the case of the amount paid for wood-pigeon shooting. This suggests that only an arbitrary cull is being achieved; arbitrary in the sense that people now do roughly the same amount of shooting each year, and claim a fairly constant and acceptable level of support. It is extremely unlikely that this reflects realistically the variable level of crop damage caused by pigeons.
It is seen that most of the annual fluctuations which occur in the numbers of any bird depend primarily on juvenile rather than adult survival. Most adult birds die not through starvation but by accident – by predation, occasional disease, and pure accidents such as flying into a telegraph wire. In general, big birds are less prone to accident; they are less likely to be caught by a predator and so they tend to have lower death-rates, but there are many exceptions. Established adults must have already experienced a season of food shortage, which they have successfully survived in competition with other individuals. It is unlikely that they will suffer in subsequent years, unless a particularly lean season occurs. In other words, food shortage may only seriously affect an established adult in one year out of many (a hard winter is one example of this). Moreover, in many cases most of the adults which die by accident do so outside the season of normal food shortage; adult starlings, for example (see here), are at greater risk of death during the breeding season, when they are busily occupied with minding their young and are more often caught unawares by predators. If a population remains stable (as in Fig. 1) but produces a large excess of young, it follows that a large number of these must die. This juvenile mortality should be seen primarily as a consequence of the young birds’ competition with the adults, whose greater experience nearly always enables them to survive better than their inexperienced offspring. Indeed, the number of young which will survive depends on how many adults are lost to make room for them, the final adjustment occurring at the worst season of the year for whatever factor is limiting adult population size. This can be food without it appearing obvious. Thus, after breeding, there exists a big excess of young although there may still be enough food to support all individuals. Accidental deaths will occur throughout this time, but it is likely that young will be most severely affected through inexperience. Eventually, and it may be gradually or suddenly, the season of minimum food supplies will arrive. If by this time there are already too many adults, then virtually all the young will now be lost as well as a few adults. If some catastrophe has occurred and no adults are available, then larger numbers of young will survive to restore the former balance between total numbers and environmental resources. But these two extreme situations will occur only rarely. The above account is slightly over-simplified, as in reality adults themselves do suffer a little from competition with their young, and the process is not completely one-sided. Removal of juveniles increases survival prospects for the adults. Furthermore, it will be appreciated that several factors influencing bird numbers may act simultaneously, so that adjustments are continuous – this is why animal populations are called dynamic.
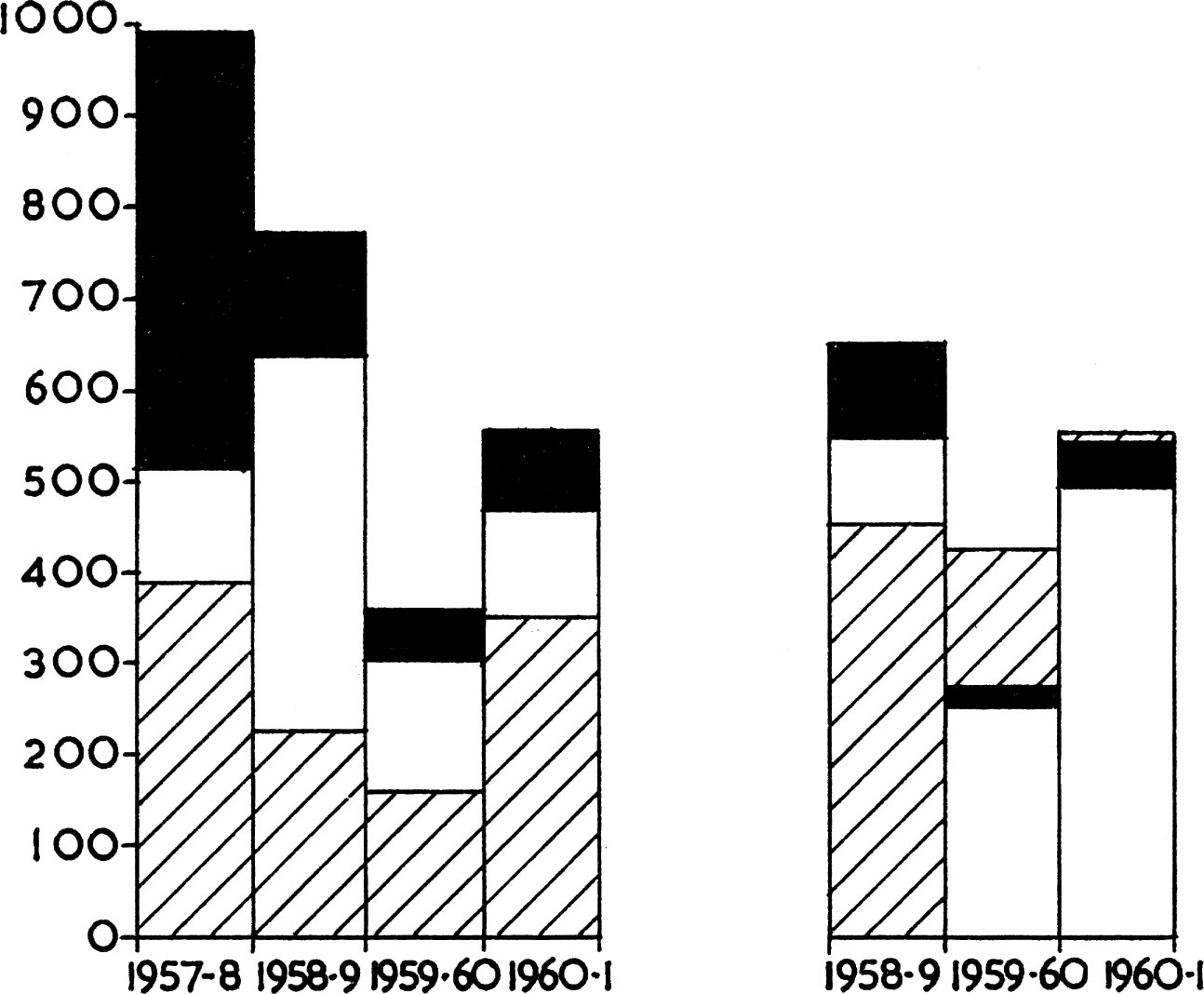
FIG. 5. The top of the columns represents the total number of grouse in different autumns on study areas in Scotland on low ground (left) or high ground (right). The number of these birds which were shot is indicated by the solid areas, the number lost through other causes by open columns and the number of grouse alive in the spring by hatching. The data show that more birds must die than are actually shot. On high ground in 1961 there were more grouse in spring and autumn, as a result of immigration. (Data from Jenkins, Watson & Miller 1963).
Our studies of the wood-pigeon provide an example of some of these processes in operation. In 1959 the post-breeding population comprised 171 birds per 100 acres with 1.3 juveniles to every adult. In 1963 there were only 101 birds per 100 acres and 1.5 juveniles to each adult. The clover food supply, at the worst time of the succeeding winters of 1960 and 1964 was near enough the same and so the population was reduced to 34 and 35 birds per 100 acres respectively. But in the 1959–60 season the competition needed to bring about balance (171 down to 34) was clearly much greater than in 1963–4 (101 down to 33). The effect on the juveniles was striking. By the February of 1960 there were only 0.1 young to every adult against 1.1 in 1964. Hence, in both years total numbers reached the same level by winter, but juveniles suffered a 96% loss in 1959–60 against 74% in 1963–4. Adult loss in the first year was 59%, and 58% in the second season. These figures do of course illustrate a density-dependent loss of young. The term mortality has not been used, because some birds were lost through the emigration of both young and old, though it amounts to mortality so far as the carrying capacity of the land was involved in mid-winter. The true annual death-rate of adults was lower than the figures quoted.
This example shows how the age structure of a population may be altered without any change in its ultimate size. The red grouse provides another illustration of this effect, achieved by deliberate killing. It has already been noted that grouse numbers in spring are unaffected by shooting. Yet Jenkins and his team found that over the autumn the death-rates of adults and young were equal (at around 70% per annum), in sharp contrast to all other birds so far studied. This was because so many birds were shot that deaths from natural causes were not fully obvious; presumably shooting is not selective of either age group. If enough animals (old and young combined) are shot natural competition between adults and juveniles can be reduced. In an unshot population of white-tailed ptarmigan in Canada (Choate 1963) the juveniles did suffer a much higher death-rate than the adults. Again, young wood-pigeons are easier to shoot than adults until mid-winter, by which time they seem to have learnt to avoid men with guns and from February onwards are no more easily shot than old birds. But they still do less well than adults when competing for food (and at breeding sites later on) and for this reason suffer a higher death-rate. Clearly, the amount of winter shooting can alter the age structure of the population without affecting final numbers in summer.
As mentioned above, before any artificial killing can result in a reduction of population size, the total number of animals killed must exceed the rate of deaths from natural causes. In addition, as increasing numbers are killed artificially, natural mortality factors cease to operate and must be replaced by artificial ones if compensatory changes are to be avoided. Inability to reach the necessary threshold means failure so far as artificial control is concerned. If numbers need to be kept down it is best, all else being equal, to defer artificial killing to the season when natural factors have taken their toll. If such population control cannot be achieved artificially and numbers cannot be held below a natural optimum, it is vital to show that any cropping does prevent damage; it is of course feasible that artificial killing will remove animals before they would normally die, and so protects crops. But every case has to be taken on its own merits, and examples of wise and foolish applications will be found in later chapters.
The level around which a population fluctuates may be subjected to long-term changes not resulting from the key factors so far considered. Blank et al. have demonstrated that the number of breeding grey partridges (which have shown evidence of a general decline during the present century) is determined by the nature of the habitat. The favoured sites for nesting are an incomplete hedge, that is, a group of separate bushes often patchily arranged on a grassy bank, or a wide grass track. Jenkins (1961) also showed that a lack of winter cover (cereal crops as distinct from tall grass provide little cover) results in the formation of larger territories, because the males can see rivals at greater distances and this causes the breeding population to be lower; under these circumstances surplus birds move on to marginal habitats. It is conceivable that changes in land usage and farming techniques have harmed the partridge by causing the population to fluctuate around a lower level.
The well-documented decline of the corncrake seems to have resulted from farm mechanisation, which has eliminated the old hand cutting of hay and enabled the harvest of silage to take place earlier, to the detriment of nesting corncrakes. Once generally distributed throughout the British Isles, the species disappeared from East Anglia before 1900, from east midland and southern England by about 1914, and from Wales, northern England and east Scotland by about 1939. It remains common only in Ireland, parts of western Scotland and the Hebrides, Orkneys and Shetland – areas where the old methods of hay production to a large extent remain unchanged.
Britain has experienced several periods of radically altered climate which must have considerably affected bird distribution, particularly of those species at the edge of their range in northern Europe. Since the mid-nineteenth century changes in air circulation have caused a warming of the atmosphere particularly noticeable in northern areas; in central England, the decadal mean temperature of the summer months had risen about 2° C between 1900 and 1950, while a similar increase occurred in Finland and elsewhere. The temperature increases were particularly noticeable further north and at first were limited to the winter months (a 9° C increase in Spitsbergen), causing arctic ice to melt and polar seas to become warmer, but leading by the 1880s to increased temperatures during the northern springs, and to a summer increase in the 1920s.
Since about 1950 there has been a reverse trend in weather conditions which can be expected to bring about another southern displacement of the avi-fauna. Another complication is that the increase in mean temperatures has, in the maritime countries like Britain, resulted in distinctly wetter and cloudier summers. This is doubtless the reason why certain birds which depend on large flying insects have declined in recent years. Red-backed shrikes still produce more than enough offspring to ensure their increase, given the right conditions, but the bird has markedly declined in Britain and other parts of north-west Europe. Destruction of their habitat has sometimes been held to explain the decrease but this is by no means always the case, many areas now untenanted by shrikes appear to be unchanged, certainly in several Suffolk and Surrey localities that I know personally. Peakall, who has documented the decline, believes that a changing climate provides the explanation as flying insects become scarce on grey cloudy days. This was brought home to me very clearly when photographing red-backed shrikes on a Surrey heath in 1967 (see Pl. 1). During a six-hour session in a fully accepted hide when it was dull and overcast, the adults were clearly finding it difficult to get food. Even though they had large hungry young, each parent was visiting the nest about once every hour, bringing mostly ground beetles, until eventually they found a nest of young birds, and for a while flew back and forth with the nestlings. The following day was bright and sunny and the adults were feeding the young every five minutes or so, bringing dragonflies, butterflies, bees and lizards – in fact all the creatures which depend on sunshine to become active.
Other birds which depend on large flying insects, and which are at the edge of their distribution in north-west Europe and Britain, seem to be suffering a similar south-east contraction in range. Monk has shown that in 1850 the wryneck was very common in south-east England and the midlands and was scarce though regular north to the borders and west in Wales. By 1954, only 365 pairs could be accounted for and the total dropped to about 205 in 1958, since when it has fallen further. Moreover, well over three-quarters of the British breeding population is now confined to Kent. According to a survey carried out in 1957 and 1958 by Stafford, the nightjar is much more widely distributed in Britain, and although most common in southern England, it is frequent in the north of England and Wales though only irregular and local in Scotland. As it feeds on night-flying insects it has presumably been less adversely affected by cloudy summers. Even so, the survey showed that a decline has occurred during this century – though increased disturbance may be an important factor with this large and quiet-seeking species.
The stonechat, a bird of gorse commons, seems to have declined for a different reason. It feeds on large insects but catches them on fairly open ground, using a convenient bush as a vantage point. The ground and low herbage dwelling invertebrates on which it feeds can be found even in winter, so it remains a resident, like that exceptional Sylvid warbler, the Dartford warbler, which locally shares a very similar habitat. Magee, in a study of the stonechat in 1961, obtained breeding records from only twenty-three counties in England and seven in Wales, mostly only small numbers being involved. Only nine English, four Scottish and three Welsh counties had more than twenty pairs, whereas at the turn of the century the species bred in every English county. In 1961, 264 pairs were recorded in Pembrokeshire, 262 in Hampshire, 150 in Glamorgan, 104 in Cornwall, 96 in Devon and 51 in Dorset, after which Surrey with 39 pairs had the next highest English or Welsh county total. Magee pointed out that counties like Cornwall and Pembrokeshire have long stretches of coastline and still have extensive bracken and gorse headlands providing suitable conditions for the species. Otherwise poor gorse-covered commons or alternatively heath moor with numerous bushes have been largely lost as a habitat in Britain; the only extensive areas are to be found in those counties where tolerably high numbers still occur. Another complication is that severe winters hit stonechats very hard and it may take several years for numbers to recover. In this connection the coastal counties are probably less seriously affected, and Magee gives evidence that following any hard winter, recolonisation is usually first noticed in coastal areas from which birds then spread to inland habitats. In recent decades the predominantly mild winters between 1917 and 1939 allowed a fairly extensive re-occupation of inland habitats after the drastic reductions of the 1916–17 hard winter (41 consecutive night frosts at Hampstead) only to be followed by extensive reductions again with the hard winters of 1939–40 and subsequently. Hard winters are not regulatory factors in the accepted sense. Blanket snow cover or extended frosts affect wide areas irrespective of the number of animals present which must all suffer in a density-independent manner. A measure of density-dependence may be imposed if the animals are able to compete for restricted pockets of food; but this is a special case.
In south-west Europe, Cetti’s warbler is one of a small group of resident warblers which are similarly sensitive to hard winters and this applies to the resident Dartford warbler which is on the edge of its range in southern Britain. Cetti’s warbler spread north in France during the 1940s and 1950s with the period of mild winters and these also enabled a large proportion of Dartford warblers to survive in winter and then spread to other suitable habitats; a similar population increase and irruption to new areas occurred in the bearded tit for the same reason. The Dartford warbler formerly extended from Suffolk and Kent to Cornwall, but fragmentation of suitable heathland at the turn of the century has virtually restricted it to the New Forest, this being the only large enough area of suitable habitat, within its range, which can provide stability. Tubbs has demonstrated how during open winters the population can build up to occupy other heathland in Surrey and north Hampshire, where too small a population exists to withstand bad winters and where it has been exterminated two or three times since the 1940s – the winter of 1947 proving particularly disastrous. Tubbs has rightly emphasised the importance of maintaining the New Forest as a reservoir for the species. Here its numbers increased from around 80 pairs in 1955 to 382 pairs in 1961. Then came the snow of 1962 which exterminated the species in Surrey (the birds were trapped while roosting in tall heather by an overnight blanketing fall of snow) and left only 60 pairs in Hampshire. The following hard winter of 1962–3 virtually exterminated even the New Forest population, as well as causing a big retreat to the south of Cetti’s warbler in France.
Apart from changes in range caused by weather, several species have recently increased in response to addition to available habitats, which are often man-made. In inland Eurasia the little ringed plover replaces the ringed plover and nests on the sand and pebbly shores of slow-moving rivers or inland lakes, predominantly near fresh water. A pair nested at the Tring reservoirs, Hertfordshire, in 1938-the first nesting record in Britain. No further nests were found until 1944, when two pairs nested in another part of Hertfordshire, and one pair in Middlesex; the increase since then is shown in Fig. 6. By 1962 there were approximately 157 in Britain, nearly two-thirds being concentrated in the counties south of the Welland-Severn line and none extending further north than Yorkshire or west of Gloucestershire or Cheshire. Parrinder, who has documented these changes, points out that gravel pits provide over three-quarters of the nesting sites of little ringed plovers; sewage farms, reservoirs, brick pits and such places comprise the remainder. Most gravel-pits are centred in the areas where the species already occurs, except that a surplus of potential sites appears to exist in Lancashire, Northumberland and Durham and parts of Wales and these sites may next be occupied. Parrinder emphasises how gravel and sand production for building and road construction have increased with the post-war building boom and, as much of the material is derived from new workings, these have also increased. There can be little doubt that the creation of a new environment has favoured the species. It is interesting that our ringed plover has not been able to colonise these places, particularly as a local inland breeding population occurs in the East Anglian Breckland and the bird also breeds inland on some of the east Suffolk heathlands and in parts of northeast Scotland, for example in the Abernethy Forest.