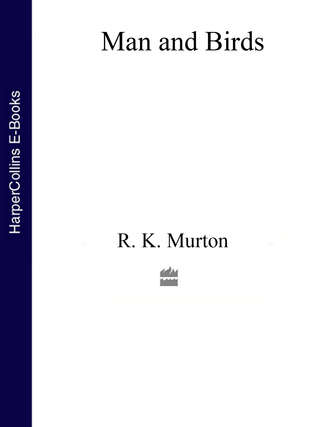
Полная версия
Collins New Naturalist Library
CHAPTER 2
ECOLOGICAL CONSIDERATIONS
A THOROUGH insight into the relationships between birds and humans demands some understanding of population ecology, a knowledge of how animal numbers fluctuate and change through births and deaths and of the factors which determine these processes. Populations have dynamic properties and these cannot be neglected by people concerned with wild life management, whether as game preservers, conservationists, pest controllers or farmers. This chapter attempts to set out some of the basic principles with pertinent examples, but these same principles will emerge in later sections in various guises. Our knowledge of the subject was first collated and clearly enumerated by Dr D. Lack (1954) in a stimulating book The Natural Regulation of Animal Numbers since when more field studies have been made by various workers, which Lack (1966) has summarised in his Population Studies of Birds. Both books should be consulted by the reader who is really interested in this subject. In this account I have drawn on examples which, wherever possible, have direct relevance to economic ornithology.
Farmers tend to the pessimistic belief that all problem birds become more abundant every year. Yet careful counts of most such species living in stable environments usually show there to be no clear tendency towards a steady increase, or even decrease, though numbers may fluctuate from year to year. For instance, many farmers believe that the wood-pigeon has increased drastically during this century to become more of a pest, though in conditions of stable agriculture this is unlikely to be true. In fact, there is evidence of a decline since the early 1960s associated with a reduction in the acreage of winter clover-leys and pastures. Certainly the species has moved into newly developed marginal land; places like the east Suffolk heathlands, which have been ploughed and claimed for agriculture since the Second World War, have been colonised by wood-pigeons. Fig. 1 gives some indication of how wood-pigeon numbers have varied on a Scottish estate near Dundee since 1887, and it is evident that fluctuations have occurred within narrow limits, with no evidence at all for a sustained rise or fall. In contrast, Fig. 1 also shows how the closely related stock dove has increased over the same period; this species first colonised in Scotland in 1866, reached Fife in 1878 and increased dramatically in Scotland in the next ten years. Every year each pair of wood-pigeons rears on average just over two young and it is evident that if all these survived to breed in their turn, population size would increase exponentially. That this does not normally occur indicates that some form of regulatory mechanism must be operating. Furthermore, this regulation must be density-dependent, that is, it must become proportionately more effective at high population densities and proportionately less effective at low ones. If the regulatory factor (s) operated without regard to density, it is evident that population size could fluctuate widely without reference to a particular level – to the constant mean represented by the dotted line in the figure.
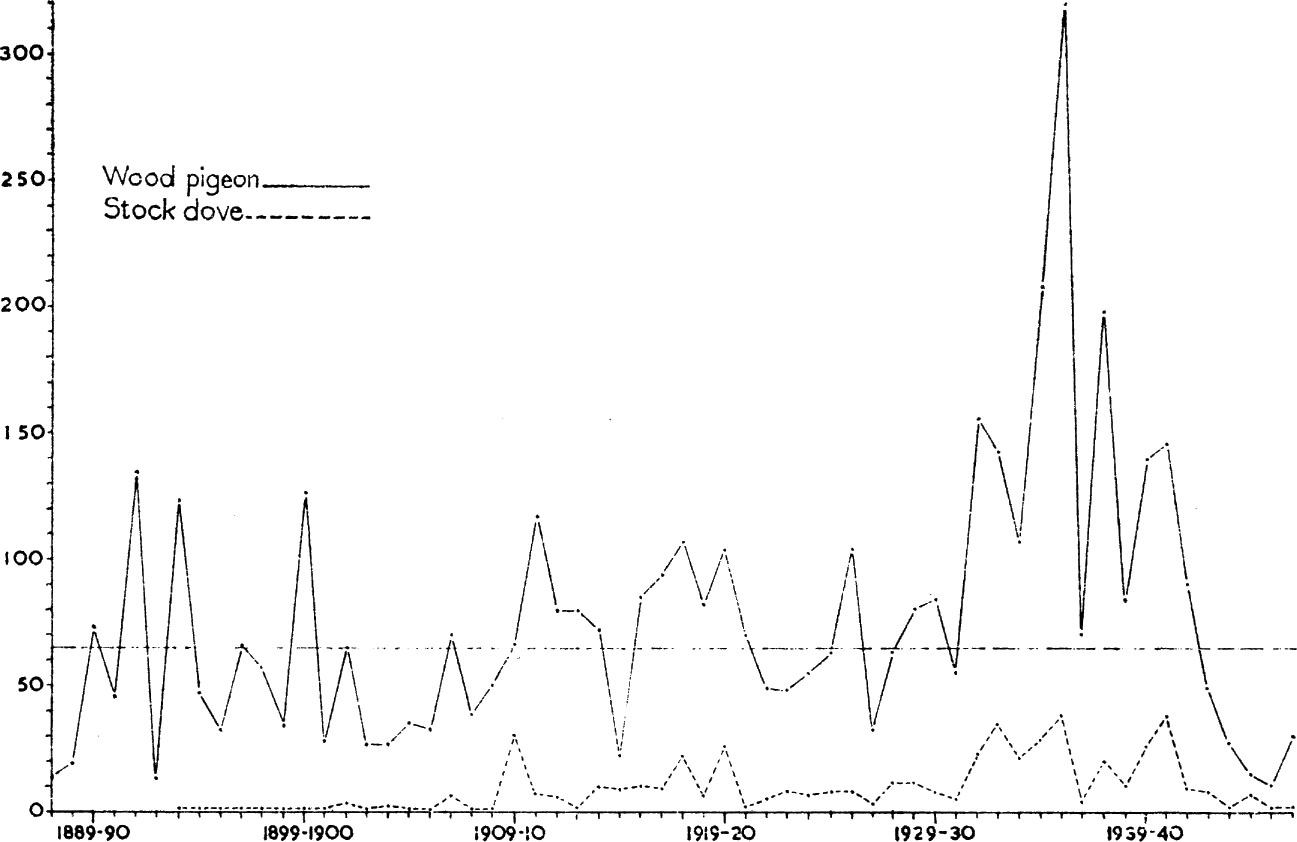
FIG. 1. Annual number of wood-pigeons and stock doves shot on an estate near Dundee. The data refer to an area which remained virtually unchanged during the period under review, and nearly all the birds were shot by one man who maintained a reasonably constant shooting pressure. They, therefore, probably provide a fair index of the total population. The autumn of 1909 was a disastrous one for the harvest owing to gales and rain from August until October so that much corn remained uncut. This resulted in an influx of wood-pigeons and the appearance of stock doves in large numbers. (Data by courtesy of Dr J. Berry.)
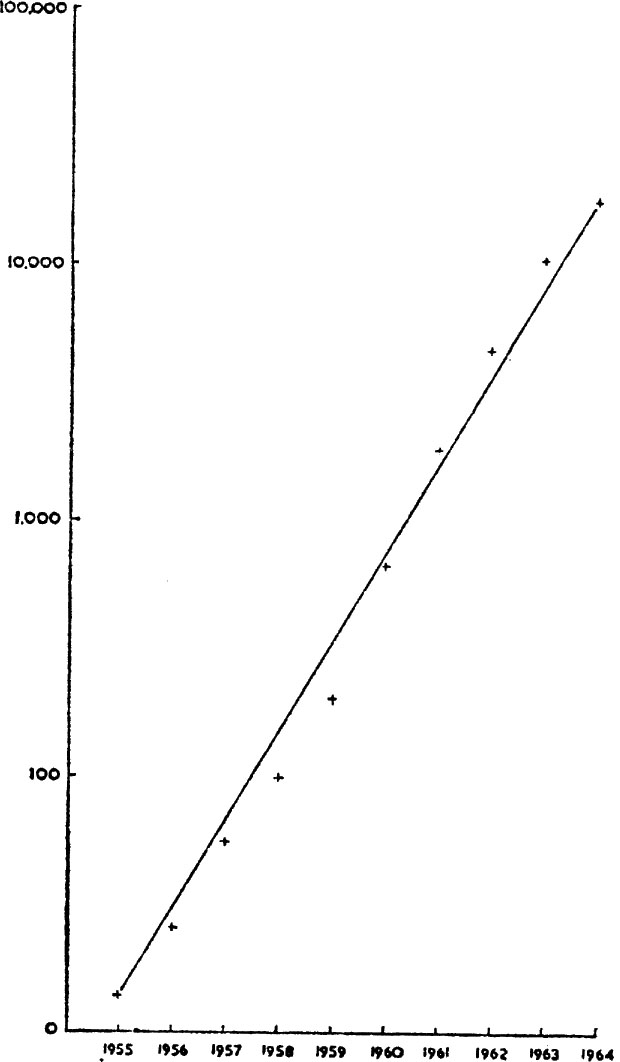
FIG. 2. Logarithmic increase of the collared dove in Britain. (Data from Hudson 1965).
Sometimes bird numbers do in fact increase geometrically, as when a species moves into a previously untenanted region where there is scope for it to live; in biological terms where a vacant niche exists (see below). In this way the collared dove dispersed dramatically across Europe to reach Britain in 1952. It had spread to south-east Europe from northern India by the sixteenth century but had then remained static in its European outpost until 1930. It has subsequently spread north-west across Europe, reaching Jutland in 1950 and Britain in 1955; an expansion 1,000 miles across Europe in twenty-four years. Why this spread was so long delayed is not clear, though it is most feasible, as Mayr has suggested, that a genetical mutation occurred which suddenly rendered the species less restricted in its needs. (We can imagine a bird, though not necessarily the collared dove, to be restricted by a temperature tolerance which a single sudden genetic mutation could remedy.) Throughout Britain and Europe a vacant niche existed for a small dove living close to man; it is even possible that the decline in popularity of the dove-cote pigeon created this niche. Whatever the explanation, a high survival rate among collared doves has evidently been possible, and their potential capacity for geometric increase has been realised: see Fig. 2, which relates to Britain. The Syrian woodpecker may be on the brink of a similar explosion. It is considered to be a recently evolved species (post-glacial) which replaces the great spotted woodpecker in south-east Asia. It spread to Bulgaria to breed in 1890, to Hungary in 1949 and to Vienna in 1951. A significant feature of the bird’s ecology in Europe is its confinement to cultivated areas which do not suit the great spotted woodpecker. If it proves to be better adapted to this man-made niche we can anticipate a continuing advance across Europe. Agricultural development in the Balkans may well bring other surprising range extensions.
Accepting* that populations are controlled in a density-dependent manner the next question to consider is the nature of these regulatory processes. Fundamentally, either the birth-rate or death-rate must be the factor of change. Wynne-Edwards and his supporters have argued that the reproductive rate is of much importance and that those animals with a high expectation of survival, such as many seabirds, have evolved low reproductive rates and vice versa. They have also claimed that a host of conventional behaviours have evolved as a means of regulating numbers. For instance, Wynne-Edwards regards the eating of eggs and young, practised by many raptors and also storks, as a device to limit their reproductive output; deferred maturity (gulls do not breed until three or four years old), territory formation, and various other behaviours are similarly regarded in this light. These views form part of his more general thesis that animal numbers in undisturbed habitats are at an optimum density and that maintaining this optimum has selective advantages. Special cases of this theme have attracted various supporters. E. M. Nicholson (1955) is one and he ascribes population control to density-dependent movements, rather than to mortality, while Lidiker (1962) goes further in seeing emigration as a mechanism enabling populations to have densities below the optimum carrying capacity of their habitat. Hence, Wynne-Edwards regards behaviours such as those listed above as homeostatic (self-regulatory) mechanisms, being induced by the population rather than by the environment.
Wynne-Edwards also drew parallels between bird and human populations, claiming that infanticide, taboos and other methods of reproductive restraint practised by so-called primitive societies were extensions of these same deep-rooted animal behaviours. In so doing he resuscitated some very early ideas of Carr-Saunders (published in 1922) which the well-known demographer did not subsequently repeat. Indeed, Mary Douglas, in criticising the idea of an optimum population, points out that there are many examples of human under-population. Considering certain primitive societies in detail, she makes it clear that they represent highly evolved and complex groups about which generalisations are meaningless. For instance, the Netsilik Eskimos live in a harsh environment where males suffer a very heavy mortality in hunting, and female infanticide is practised primarily to maintain an even sex balance. The Ndembu, a Lunda tribe in Zambia, grow cassava as their staple crop and live at a density of 3–5 people per square mile, whereas cassava could support a density of 18 people per square mile. The reason for the discrepancy is that the tribe passionately love hunting and move their villages to where game is available so that they never reach a stage where they are up against the ceiling imposed by their basic resources. As Douglas says: ‘they live for the oysters and champagne of life not the bread and butter.’ On the other hand, the Rendille are a tribe of camel herders in Kenya and live on the meat and milk of their sheep and camel herds. An optimum number of people is needed to maintain the camel herd, and when smallpox reduced man-power, stock had to be lost. In more normal conditions a balance of man-power is achieved by emigration, monogamy (herds are not divided but go only to the eldest son), while a measure of infanticide is practised (all boys born on Wednesdays).
In general terms, homeostatic population control in human groups depends on limited social advantages (the enjoyment of hunting by the Ndembu; education, motor cars and social prestige in western Europe) and not on any relationship to resources per se, as is the case with infra human species. Douglas could well be right in not attributing the increase in the Irish population between 1780–1840 to the adoption of potatoes as a staple diet, but rather to the ruination of Irish society by penal laws and English trade tariffs. Similarly she argues that the miseries of enclosures and the Poor Laws resulted in the population explosion which led to the Industrial Revolution, rather than that increased resources stimulated an increase in population. If true, this is quite different from the way in which animal populations are determined.
Animal populations cannot be directly compared even with the supposedly most primitive of human ones. Moreover, unequipped with any cultural tradition and means of communication, some special mechanism would have to be found to account for what amounts to altruistic behaviour. The Darwinian viewpoint is that if two animals (of the same or different species) are in competition, the one able to maintain the highest reproductive output must succeed at the expense of the other, all else being equal. This is the meaning of natural selection, which amounts to individual selection. What then would prevent the genes of parents which practise restraint from being at first swamped and then lost in competition with less socially inclined individuals? Wynne-Edwards surmounted this problem of explaining how individuals could acquire genes which cause them to behave in socially advantageous ways, sometimes at their own expense, by invoking the concept of group selection. In other words, in many situations survival of the group is more important than survival of the individual. Without entering into detail it must be said that the genetical basis for group selection is very restricted, and it can only be shown to be feasible in small isolated populations (birds do not satisfy the requirements of isolation as envisaged by geneticists) or in those, such as the social insects, where numerous genetically identical individuals are produced from one female, for which the term kin-selection is more appropriate. It seems to me that all the examples given by Wynne-Edwards can be answered (or will be when knowledge accumulates) more satisfactorily by individual selection and that to introduce group selection is unnecessary. This applies to two situations which I have studied closely – the peck order in birds and stress disease. I myself, therefore, reject the concept of an optimum population in this sense, together with the view that animals impose their own control over population increase. I have discussed the subject at some length because it defines my approach to the subject of applied ornithology. So far as reproduction is concerned I fully support Lack’s thesis that the reproductive rate has evolved as the highest possible; selective disadvantages follow from the production of more or fewer young than the optimum number. Disadvantages which accrue from overproduction include reduced survival chances for the offspring because they are undernourished, or impairment of the parents’ health; underproduction leads to a failure in intra-specific competition with more fecund individuals.
As most birds produce a large excess of young, the total post-breeding population increases considerably, two-fold in the wood-pigeon, up to six-fold in the great tit, but by less than half in the fulmar. Various mortality factors, including disease, predation and starvation, now remove surplus individuals so that a balance with environmental resources is achieved, and a pattern of sharp fluctuations within each twelve-month period is superimposed on more subtle changes in the breeding population from one year to another. Lack has argued, and again I agree with his views, that the food supply is usually, but not invariably, the most important factor affecting these annual fluctuations in birds. While food could ultimately be the most important factor in all cases, other agencies, for instance predators, may hold numbers below the level which would be imposed by food shortage. The most important fact to appreciate from the viewpoint of economic ornithology is that causes of death are effective until the population is in balance with the environment, and in the absence of one such factor another will take its place. Conversely mortality factors are not usually additive – in the sense that two together do not decrease numbers more than one alone. The degree of stability seen in a population will, therefore, depend primarily on that of the environment and not on any essential characteristic of the population. By environment we not only mean the food supply but include all the other components, biotic and physical, which may interact to cause competition and mortality, directly or indirectly.
Blank, Southwood and Cross have recently shown in a neat but semi-mathematical way how the various causes of mortality contribute to the regulation of a partridge population on a Hampshire estate which was studied from 1949–59. I shall illustrate less elegantly certain aspects by using as an example another partridge population living on a Norfolk estate, for which details of annual fluctuations in numbers have been given by Middleton and Huband (Table 1). Following breeding, numbers increase over tenfold but there immediately follows a period during which it is mostly the young which are lost, so that from a post-breeding average of nearly ten chicks to each adult the ratio falls to only 1.5 per adult by August, most of this chick loss occurring in the first few weeks of life. Jenkins (1961) had earlier claimed that this heavy loss of young, which is particularly heavy in cool, wet and windy summer weather, was the main variable determining the number of partridges later available for shooting. This was confirmed by Blank et al., who emphasised chick loss as the major contributor towards the total mortality occurring each year in partridges, and as the most important determinant of the September ratio of old to young. They found that about half of the variation in total mortality was due to fluctuations in chick loss, but that about half this chick mortality was unrelated to the size of the population at hatching. This means that the survival of chicks was partly responsible for regulating the autumn population (in the sense that autumn numbers were largely governed by how many young survived). However, because this survival was only partly determined by population size there was a margin of production which caused autumn numbers to fluctuate partly independently of population density. Thus 35% of the year-to-year fluctuation in the September population resulted from variations in chick mortality. In further studies Southwood and Gross were able to show that 94% of the variation in breeding success (they used the ratio of old to young birds in September as a measure of breeding success, this also being a measure of chick survival as explained above) could be accounted for by variations in general insect abundance in cereal and forage crops in June. They measured insect abundance by the use of suction traps and showed that the insects sampled were in the main those eaten by partridges, judged by analysing the crop contents of chicks.
The number of partridges finally surviving to breed in March depends on mortality factors operating in the winter, shooting being the most important. Shooting is contrived to operate in a density-dependent manner with proportionately more birds being shot when numbers are high, but this is, of course, an artificial situation which masks the effect of natural regulatory agents. These last are likely to reside in the nature of the environment, the amount and type of cover which in turn influence territory size and may result in surplus birds emigrating. They are considered in greater detail below (see here) as they are involved as factors determining long-term changes in population size as distinct from annual fluctuations. To summarise, variations in chick survival dependent on arthropod food supplies are responsible for the marked ups and downs of partridge numbers from year to year. Density-related variations in chick survival, together with density-dependent winter losses, are responsible for keeping the spring breeding population within relatively narrow bounds from one year to another. Nevertheless, there has been a general long-term decline in this level which we shall consider below. It is to be noted that pre-hatching factors that influence the viability of eggs (the ability of the female to lay down yolk reserves could depend on spring food supplies and influence the viability of any eggs she laid), the hatching success of eggs, or any other cause of mortality, were not related to population size nor to variations in total mortality.
For the figures given in Table 1 it can be ascertained that the number of adults breeding in any one year was not at all related to the number breeding in the next year. In other words, a small breeding population could be followed by an increase or decrease in the following season and vice versa. But, as Fig 3a. shows, the percentage change in breeding population from one year to the next was positively correlated with the autumn ratio of young/old, this in turn depending on the survival of young during the summer. In Norfolk this post-breeding chick loss was not correlated with the size of postbreeding population. Thus for two quite separate populations of the grey partridge the major cause of changes in numbers from one breeding season to the next has been the death-rate of young in the summer months; when this has been low, breeding numbers have tended to increase. Annual differences in reproductive output have not contributed to the changes. In the Hampshire study the summer loss was dependent on the total partridge density, and this supplied the necessary regulation to keep fluctuations within relatively narrow limits. In addition, as we shall find, both populations experience density-dependent losses in winter, but the absence of any density related loss in summer among the Norfolk birds could be associated with the long-term decline this population is experiencing. (Fig. 26, see here.) However, this last suggestion needs corroboration.
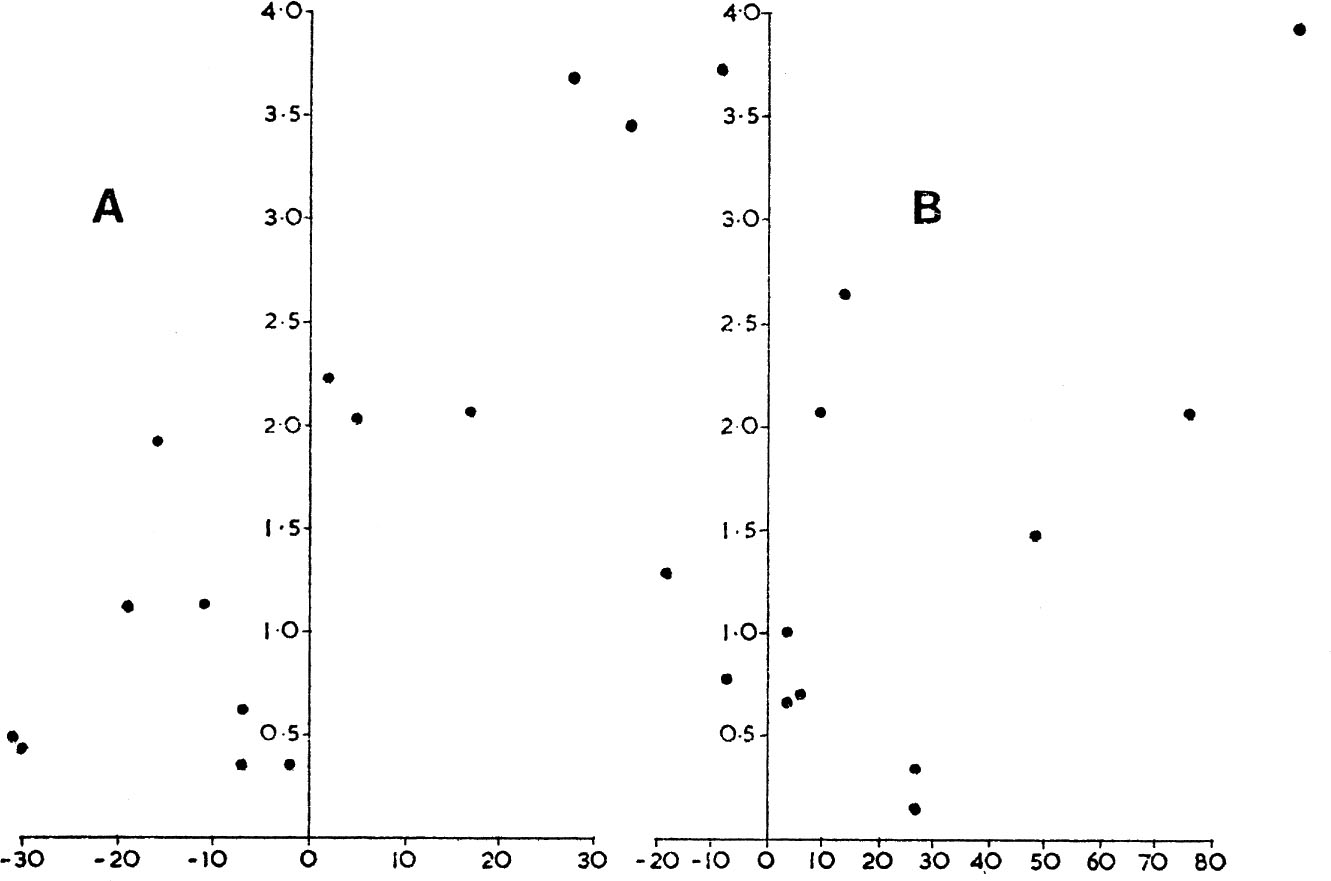
FIG. 3a. Percentage change in numbers of grey partridges between successive breeding seasons (abscissa) related to the corresponding autumn ratio of number of juveniles per adult (vertical scale). The correlation coefficient is statistically highly significant with r11 = 0.822.
3b. Percentage change in numbers of red-legged partridges between successive breeding seasons (abscissa), related to the corresponding autumn ratio of number of juveniles per adult (vertical scale). The correlation coefficient is not significant with r11 = 0.367. (Data derived from Table 1, from Middleton & Huband 1966).
Those mortality factors which, like chick loss, affect the size of the actual population, have been termed ‘key factors’ because they provide the key to predicting future population size, and are responsible for the year-to-year fluctuations in numbers. Perrins’s work on the great tit and my own studies of the wood-pigeon had earlier demonstrated that, in these two species, the major factor influencing changes in numbers from one year to the next is the survival rate of young after the breeding season, not the reproductive output itself nor the adult loss. Juvenile survival has been proved to depend on the food supply in the case of the wood-pigeon, and there is good reason to believe that it is also involved in the case of the great tit. However, for both these species, and unlike the Hampshire partridges, juvenile mortality is not seen to be density-dependent (at least not with the data so far available) and so some other cause of death could be responsible for regulating the populations, in the strict sense of the term. Lack suspected, for the great tit, that winter losses in relation to food supply may provide the critical density-dependent mortality, but emphasised the difficulty of proving this point. The problem is that food stocks and bird numbers vary considerably in different years. It may happen that if food stocks are high, bird numbers can be already low or high so that in neither case is any compensatory mortality required. It is difficult to isolate such effects, because in field studies it is not feasible to examine a variable number of the animals in relation to the same food supply each year. Failure to obtain the required data does not in this case invalidate the theory. It should be mentioned that a population will be regulated even though most of the deaths which occur are not related to density, provided that a small density-related adjustment is eventually applied. For instance, 6o%-8o% of losses could be suffered quite at random provided that after these had occurred there was a small loss, say in the order of under 10%, which was related to density. Indeed, this is the more usual situation.
On the Norfolk estate under discussion, red-legged partridges have increased over roughly the same period that the grey have declined (see Fig. 26 and Chapter 7, p. 176). It is interesting that, in contrast to the case of the grey partridge, the autumn ratio of young to old has not been the factor which determines the subsequent spring population of the red-legged partridge (Fig. 3b). There were some years when more red-legs were shot in the autumn and winter on the estate than were actually known to be there at the beginning of the shooting season, and numbers sometimes were higher in spring than in the autumn. Clearly, something has been happening which has enabled immigrant red-legs to move into the area from surrounding farms or marginal land (see here). This suggestion highlights another facet of population control which must be considered, namely the role of immigration and emigration. In the case of the wood-pigeon, juvenile birds which are surplus to the carrying capacity of the area in the autumn do not necessarily die but may emigrate to other areas – to marginal habitats or even to France. A proportion survive to return in the spring. Although winter numbers may be directly related to the food supply – for the death-rate depends on how many surplus birds exist in relation to this food supply (in a poor year for food there is not necessarily any mortality if the population is already in balance with food stocks) – the number in spring will depend not only on local survival but also on how many of the emigrants survive to return. These complications do not invalidate the contention that in any area at the worst season numbers are regulated by the food supply (for the rest of the year there may be more food than birds to eat it, especially if breeding cannot be accomplished quickly enough), but they add to the difficulty of demonstrating clear-cut relationships, and are sometimes introduced in ill-conceived arguments as evidence against the theory.