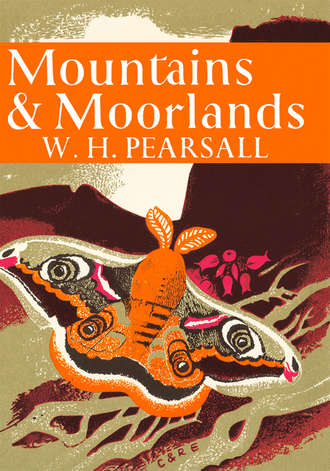
Полная версия
Mountains and Moorlands
In practice, the most widespread influence of altitude is the change in the character of the prevailing plant communities, with all that it implies in its effect on animal habitats. Most noticeable is the disappearance of woodlands and trees with their varied faunas and ground floras. As this commonly takes place at about 2,000 ft. and as the restricted montane species appear above that level, we may take it as a convenient altitudinal separation of montane and sub-montane zones.
Within the limits thus defined by temperature other factors must play their part. Every naturalist knows that shelter from wind is often vitally important, so that here and there among the mountains there are oases in which the frequency of plant and animal life is altogether different from that found on the exposed and wind-swept faces. Within the limits imposed by temperature, humidity also exerts its restrictions, not only by presenting a range of habitats running from pool or rivulet to desiccated rock, but by influencing the character of the soil. It is to the consideration of these soil conditions that we must now turn.
CHAPTER 4
SOILS

THE second important group of factors in upland habitats is the nature of the soil covering—or perhaps more strictly, of the surfaces available for plant growth. Geologically, as we have seen, these surfaces may be classified either as stable or unstable, depending on whether they are still subject to active erosion or not. As habitats for plants there is a more profound difference between these two classes. Most of the unstable surfaces are rocky or are covered by rock fragments in various stages of disintegration, and even their physical properties differ greatly from those of fertile lowland soils. They are, in fact, soils in the making, and it is one characteristic of upland areas that they exhibit in profusion all the varied stages of soil-formation. We see the native rock breaking down under the action of frost and other weathering agents to rock fragments, which become progressively finer as the process is longer continued, ultimately to yield the small mineral particles which form the basal material of most soils. The weathered material may remain in situ, covering the original rock surface, or it may be removed by erosion and redeposited elsewhere by streams and rivers as banks of silt or alluvial plains, by solifluction or rain-wash, or formerly in Britain by widespread glacial movements.
The raw mineral material is, however, comparatively sterile. It is converted into what we call a soil partly by chemical modifications resulting from the presence of water, often charged with carbon dioxide or humic acid, and partly resulting from the gradual accumulation of organic materials derived from plant remains. This latter material is called humus, and is particularly important because it forms a medium upon which can grow various micro-organisms, mainly bacteria, moulds and protozoa. With the accumulation of humus and the gradual colonisation of the material by these organisms comes a final stage, when it is usual to imagine that the original particles of mineral substance have become covered by a jelly-like mass of colloidal material—in part gelatinised minerals but also including humus—on and in which the population of soil micro-organisms lives.
It will be evident from this brief summary that upland soils can usefully be considered as belonging to a developmental series. But it is true of any soil that one of its outstanding characteristics is its capacity for change. Soils are inherently dynamic systems even when they are developed in physically stable situations, and to a far greater extent is this true of mountain soils, most of which are of geologically recent origin, even if not physically unstable.
Five types of environmental factor control the development of a soil mantle. First comes the nature of the rock or other parent material, from which soil is formed by physical and chemical weathering. Climate also exerts a marked effect on the weathering process, affecting both its physical and chemical parts, and, in particular, determining the amount of rain-water percolating through the soil in any season, a process known as leaching, which is responsible for the removal of soluble substances, bases like lime as well as plant nutrients like nitrates. Relief influences the lateral movement of percolating water down a slope, the degree of drainage and the stability, and thus affects the degree of leaching. But none of these effects is instantaneous and so there is a time-factor to be considered. Lastly, there are the obvious biological factors, of which the action of vegetation is most significant. Vegetation derives part of its sustenance from the soil and so incorporates a portion of the soil material which is returned to the soil on the decay of the plant tissues. The fertility of a soil is the result of this cyclic exchange. An efficient type of plant which draws heavily on the soil nutrients keeps them in a form of biological circulation which mitigates the losses due to leaching. Thus there is a natural mechanism for maintaining soil fertility, which, by drawing on the deep layers of the soil, is capable even of increasing the fertility of the surface layers provided the leaching factor is not too intense. Further, in any environment where the climatic factors have remained reasonably stable for a long time, it is possible for the soil-vegetation system to achieve a measure of temporary stability. In upland Britain, however, the soils are generally in dynamic states moving along definite trends of soil development. The trends due to a severe climate are particularly marked, and they operate during the different stages of soil development in the following manner.
SKELETAL AND IMMATURE SOILS
The initial stages of soil development in which rock fragments predominate are what we can only call skeletal soils. They are found principally where the surface is unstable or where further development is retarded by hard rocks or low temperatures. Of these factors, the low temperatures have also distinct qualitative effects, because while they greatly retard chemical modifications, the associated physical disintegrations caused by frost and solifluction are especially vigorous. There may thus be much physical commination of rock fragments with little chemical change. Thus the soils, even if finely divided, are immature in the developmental sense because of the deficiencies in their chemical and biological equipment. Soils of this general type occur on mountain-tops, where they are found under the mountain-top detritus except, perhaps, where it is especially coarse and deep. Generally, however, the detritus seems to be a superficial layer of stones extruded from below during the frost-caused or solifluction movements of the materials. Beneath the stones there is commonly a sandy loam, generally brown in colour and little leached. When vegetation is present this merges at the top into the almost black humus that collects among the surface detritus. The depth of the soil varies with the nature of the rock and the degree of erosion, but it is usually between one and three feet, and then comes disintegrating rock.
Parallel to these summit soils in general quality may be the scree-slopes of finely-divided material which occur lower down a mountain, often approaching stability but still subject to soil-wash and soil-creep, and so often distinguished as creep-soils. These show great variability in detail, but, like the mountain-top detritus, they often show coarse material at the surface and finer below. As they approach stability, they merge into the woodland soils described below, but in the earlier “gravel-slide” stages, a vertical section usually reveals a sequence of more or less alternating sandy or stony layers parallel to the slope (see Fig. 8). All soils of these types are alike in possessing a high base-status because they consist mainly of rock particles as yet not greatly modified by chemical change.
In all upland habitats there are in addition the overall trends caused by continual washing by rain, and as a result every exposed and porous surface will be more or less leached. Wherever leaching has taken place there must be corresponding areas that receive the products of leaching. The water that carries away lime or other bases from the higher upland surfaces must produce elsewhere lime-rich or base-rich habitats. Areas of this latter type may be distinguished as flushed or enriched habitats to distinguish them from the leached or impoverished ones.
FLUSHED SOILS
In general, of course, leaching will preponderate in upland regions and enriched soils will be commoner in lowland regions. Nevertheless enriched soils are always to be found occupying characteristic localities in mountain areas. Thus there is a flushed area around every springhead and around every rivulet. However, the water need not emerge as a separate spring but may perfuse the surface soil—a type of flush that can be recognised by a zone of greener vegetation. The various types of “damp flush” may be associated with a soil of almost any physical category. Enrichment by water from a higher level is greatest when such water has penetrated into the rock by means of structural fissures, and permeated the rock strata on its way down; a mere receiving area for surface run-off from acid upland soils is often as severely leached and as acid as the upland soil itself.
Parallel with enrichment by water (“damp flushes”), there is enrichment by presence of freshly-weathered rock particles, and areas of this type might be called “dry flushes.” The lower part of any steep slope is constantly enriched by such particles washed down from above. Screes and gravel-slides, in which the breakdown of new rock by weathering continually yields a supply of bases, could thus be considered among the enriched or flushed habitats. In this category also comes any unstable surface, crag, gullies and the like, where new rock surfaces are being exposed by erosion.
It will be observed that the flushed habitats are determined by a diversity of factors producing enrichment and have thus few physical characteristics in common. They tend to fall technically into four categories:
1 Bare rock or oversteepened slopes with soil particles washed away or present only in narrow fissures. Enrichment by continual weathering of freshly exposed rock surfaces.
2 Block scree in which leaching tends to preponderate over weathering, although the latter nevertheless does continually refurnish some of the bases lost, especially in the case of more rapidly weathering and base-rich rocks.
3 Unstable scree-slopes and solifluction areas with movement and accumulation of weathered soil particles, often below the surface layer of coarse detritus; enrichment both by weathering and particle accumulation.
4 Accumulation areas—nearly always showing fine and deep soils, with enrichment mainly by accumulation from above.
Upwelling of base-rich waters may occur in conjunction with any of the four categories above, although it is rarest in a and commonest in c and d. In both c and d the total “flush” effects normally counteract losses by leaching unless the soils are deriving from base-poor rocks.
LEACHED SOILS
On the whole, the unstable surfaces are areas of enrichment, and as such show distinctive types of vegetation. When they become stable enough to permit a complete vegetation cover, they inevitably tend to become leached—a tendency that is in some slight measure accelerated or retarded according to the nature of the plant cover. Because heavy rainfall is the rule and because plant remains tend to accumulate on the soil surface, it is characteristic of upland soils, once soil-creep and the forces of erosion are sufficiently arrested, that they rapidly develop the stratification or “profile” indicative of the more advanced (or “mature”) stages of soil development.
The extreme form of stratification found is that of the soil type known as a podsol, in which, under a surface layer of peaty humus and of humus-stained soil, there is a grey soil-layer from which almost all of the available bases (especially lime and iron) have been removed by leaching. With them also have gone the finest particles of clay and the humus colloids. These accumulate at a lower level, commonly two or three feet below the surface, as a dark-brown layer of “humus pan,” while immediately below this can usually be seen a red- or orange-brown precipitate of iron compounds (“iron pan”). Still lower is the little altered parent material. Thus these highly leached soils show a characteristic soil profile, with the following layers:
A. a Surface peaty humus and “litter”
b peat-stained inorganic soil
c leached grey ” ”
B. d humus accumulation zone (“pan”)
e iron ” ” (“pan”)
C. f little altered parent material
In continental areas, podsols characterise the cold temperate climates on stable and porous substrata receiving moderate rainfall. They are there associated with the northern evergreen forests of coniferous trees (firs) and with an abundance of small shrubs like the heathers.
In the British uplands, podsols are most characteristic of the eastern regions where the annual rainfall is relatively low (say about 35–40 in.) and the parent material is often sandy or gravelly morainic material. They are often now associated with heather-moor and pine forest, and originally this association may have been still more widespread. In the wetter western areas, although podsolic features in the soils are frequent, good examples of podsols are infrequent. Often this is because of great irregularity or diversity in the composition of the parent material. In particular, the deposits of iron or of peaty colloidal matter which suggest the appearance of a podsol are often due to lateral seepage from higher up the slope and are not necessarily derived from the soil-layers immediately and vertically above them. Varying porosity, in particular, leads to very irregular local accumulations of the humus and iron colloidal layers, which may appear in blotches along the lines of seepage and at a varying distance below the surface. But it is probable that most of what were once free-draining forest soils have now been transformed to bog (see below and Chapter 10), and that those which are left are but transitional stages.
Between the extreme podsols and the young or slightly leached soils, there is a range of soil profiles usually classified together as “brown earths” from their ochreous brown colour, and in the lowlands characteristic of forests of deciduous trees, oak, beech and the like. The surface layers have been somewhat leached of bases but retain a brown colour along with a base-status sufficient to give a moderate fertility. This is the characteristic soil-type of lowland Britain. In the uplands, however, this condition can only be long maintained, where the original rock fragments are base-rich, where flushing of some sort maintains the base-supply, or where the vegetation is such as to renew the base-supply in the surface layers. The latter condition would be favoured, for example, by oak woodlands rather than by a covering of birch or of pine, for the lime contents of the leaves of these trees differ considerably: that of oak approaches 3 per cent, while those of birch and of pine are only about 1·5 and 1·0 per cent respectively. Thus by absorbing more lime from the lower layers of soil and returning it to the surface, oaks would maintain the base supply of the surface soil-layers for a longer time and so would tend to retard the effect of leaching.
The upland soils of brown earth type are usually recently stabilised “creep soils,” flushed glacial drifts, or soils derived from base-rich rocks. Almost always they were until recently under woodland, normally of oak though often with much ash. Now they are almost always cleared of trees and are covered by grasslands of various types. The removal of the original tree-cover was often followed by destruction of the surface humus through its oxidation and by the removal of the surface layers by rain-wash. Thus many upland soils of this general type, like the “frydd” soils in Wales, have been considered now to show “truncated profiles,” the top strata having been removed, often by erosion. In some cases, however, it is also probable that the profiles are immature and that the soils owe to this their comparatively high base-status.
It is quite clear that generally in the uplands the process of leaching can only be retarded and not completely stayed. The high summer rainfall in particular ensues that leaching will continue under the most favourable conditions of temperature. In lowland climates, in contrast, there is in summer an excess of evaporation and drying in the surface layers of soil so that base-rich water ascends from below by capillarity. This opportunity for replenishment is lacking even in a well-drained upland soil so that the soils as a whole must tend towards the leached condition.
Thus it seems inevitable that any porous soil, once stabilised, must ultimately develop towards a podsolic stage. In the majority of cases it seems that the process has not ceased at this stage. The downward movement of fine particles of clay and humus which characterises podsol development leads to the formation of impermeable pan-layers which impede drainage. Thus under conditions of high rainfall the upper layers of soil become waterlogged, seasonally if not permanently. This leads to peat accumulation, which in turn accentuates both leaching and poor drainage. Thus in mountainous Britain, podsols have almost always tended to become peat-covered bog soils, such as are described below, and it seems probable that the characteristic podsolic profile becomes modified when this change takes place, and ultimately disappears.
These trends of soil development favourable to bog formation are undoubtedly much accentuated by the topographic relations of the mountain soils. Once bog conditions have been established at any point on a hillside—a process which in most cases clearly must have taken place at an early stage in the physiographic history of the area—there must inevitably be a tendency for bog seepage to extend downward and to produce a general degeneration in the soils below it. The greater the area of peat accumulation in the upper bog zone, the more rapidly would this influence extend downwards. Moreover, because a mountain is a gathering ground with a considerable excess of rainfall over evaporation, it seems likely that the lowest slopes will often also show a marked trend towards the establishment of bog, the degree to which this happens being controlled by the geological structure of the mountain and the amount of mineral bases (lime, potash and so on) the mountain yields to the seeping water. So soon as the mountain-side becomes stable enough to show signs of leaching these trends are strongly in favour of it passing over to bog. Thus the upland soil problems are very complex, affected not only by the immediate characters of the soil but by lateral movements, by adjacent relief, rock and vegetation.
CHEMICAL STAGES IN LEACHING
The chemical stages which can be distinguished during the leaching process can now be outlined. In the first instance, most British soils are principally calcium soils, or, putting it in another way, they have lime as their principal base, and are lime-saturated. Agriculturally and ecologically, soils of this type possess high fertility. As leaching goes on, most of the lime and other bases are removed, being replaced by hydrogen, so that the soil finally becomes acid and sour (e.g. to the taste). For ecological purposes there are, however, two intermediate stages in this process which can usefully be distinguished: a state of partial lime-deficiency and one of higher lime-deficiency. Most British soils also contain a good deal of iron oxide—to which their colour is largely due—and under conditions of good aeration this is removed much more slowly than lime. The following four types of soil can conveniently be recognised as stages in the leaching process:
1 Lime-saturated;
2 Lime-deficient;
3 Base-deficient, iron oxide becoming mobile and relatively more important than lime;
4 Acid, with podsolic profiles in stable soils, often masked by peat accumulation.
Of these b and c represent the stages usually referred to as brown earths. The ecological value of this series lies in the fact that very decided transitions in vegetation occur at a point between b and c (which is also approximately half-way between a and d)—that is, a point of half-saturation with bases.
The technical methods of distinguishing these soil-types depend on the methods of measuring the percentage of base-saturation, which decreases from a to d, or of measuring the increasing acidity. The latter is perhaps a mode of expression more familiar to biologists. Estimates are made of the hydrogen-ion concentration and expressed in the following way. Concentrations, varying, for example, as 1/100, 1/1000 or 1/10,000 gm. per litre of hydrogen ions can be written either 1/102, 1/103 or 1/104, or 1 x 10-2, 1 x 10-3 or 1 x 10-4 g/l, and for convenience these are termed pH 2, 3 or 4 respectively. The notation extends over a range of 1 to 14. In terms of this notation, pure water has a pH value of approximately pH 7, lime-saturated soils have a somewhat similar pH value, of above 6, while natural soils which are about half-saturated with bases have a pH value of approximately 5. The characteristic acid soils in the ecological sense lie below pH 3·8.
These soil types can, however, often be distinguished by their appearance and biological characters. The grey and leached zone in a well-developed podsol is likely to be mainly a hydrogen soil, as is the humus-stained layer on the surface. In many upland soils, the leached but still brown layer of inorganic materials below the surface humus has a characteristic orange-brown colour—not grey as in a proper podsol. This condition is associated with the removal of most of the lime and the mobilisation of iron, at first perhaps dissolved from the soil minerals near the surface by humus compounds, but then reoxidised on the surface of the soil particles in a state which accounts for the characteristic colour. This type of soil is probably very definitely associated with periods of waterlogging, such as are frequent in upland areas, and it normally has a lower base-status than the more typical soils of brown-earth type.
In these and in other ways, therefore, the characteristic appearances of soil profiles give a good deal of information about the base-status of the soils. It is perhaps worth emphasising also at this stage that two factors in particular are especially effective in removing iron and other bases during the final stages of the leaching process.
One of these is the increased acidity and especially the effects of acids derived from plants like oxalic and citric acids, in which iron salts are especially soluble. The second is the establishment or development of waterlogging, which, by eliminating the oxidising effects of air or oxygen, permits the reduction of iron to the ferrous state, in which it is very much more easily soluble, as well as more readily replaced by the process known as base-exchange. In aerated soils, however, waterlogging can only be temporary even though it must be frequent in winter in all upland soils. When it occurs permanently the soil commonly acquires a blue-grey appearance which we associate with the presence of ferrous iron compounds, and this contrasts very noticeably with the reds and browns of the ferric salts in air-containing soils.