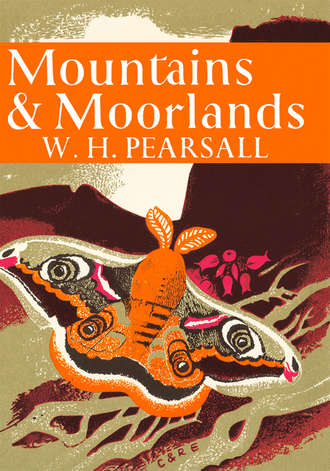
Полная версия
Mountains and Moorlands
In attempting to summarise what has been obtained from this survey, it becomes clear that physical instability is the most noticeable feature of upland surfaces, and it is equally evidently a chief characteristic of the high-level or montane region—although it also accompanies any steep slope as well as the borders of active erosion systems such as streams. Physically stable areas in the uplands differ little from lowland areas, except in other features such as those of climatic origin.
We also see that British mountains are often likely to show an upper zone of comparatively gentle slopes, representing the ancient land forms, moulded long ago, but often kept alive or unstable through the agencies we call nivation. The lower slopes have often been over-steepened in comparatively recent times as a result of glaciation or of the extensive erosion which must have been associated with the melting of the ice. This common plan, if we may call it so, results in the appearance of numerous rather round-topped mountains, although it is modified in innumerable ways as a result of the varieties of rock which make up the mountain blocks and of the different sorts of bedding planes which may be found in different areas.
There is still another way of looking at these matters. The present cycle of erosion as it affects the upland surfaces may be considered to have started at the end of the Ice Age. The upland surface at that time, except where covered by drifts or morainic materials, must have been very different from what it is to-day. It must have been mostly exposed rock which, presumably under the sub-Arctic post-glacial conditions, quickly developed frost-shattering and the characteristic erosion forms found to-day in the Arctic and at high altitudes. To-day much of the corresponding surface is soil- or peat-covered, and only the montane or unstable areas preserve what must have been a widespread condition in the immediate post-glacial period. It will be seen, therefore, if this argument is correct, that the study of the montane areas is likely to be of especial interest. We shall expect to find that the biological character of the unstable areas is widely different from that found elsewhere and perhaps in some respects reminiscent of a condition that was more widespread in post-glacial times.
CHAPTER 3
CLIMATE

THE differences between upland habitats and those of the lowlands are only partly structural. Partly they are climatic and this aspect must now be considered. British mountains are only of moderate size but they lie near the sea and across the path of the strong Atlantic breezes from the west. For this reason, wind and cloud and rain play a large part in the weather conditions and they combine to give a characteristic “atmosphere” to British mountain scenery, something of which is conveyed in the photograph of Glen Einich in Pl. VIII. Equally familiar to inhabitants and noticed by many visitors is the building up of evening cloud after sunset (see Pl. XXIX), while even in the finest weather the day is likely to break beneath a curtain of morning mist, well shown in the charming photograph of Llyn Padarn, (see Pl. 10). The visual impressions we thus carry with us can readily be confirmed from the precise data collected by meteorologists, and to them we may now turn.
We are fortunate in having detailed records which enable us to assess these effects over long periods and thus to present them as the main features of mountain climate in Britain. They were made between 1884 and 1903, when an observatory was maintained near the top of Ben Nevis (4,406 ft.), and though they thus give the extreme climatic limits for British mountains, they enable other more scattered observations to be checked and utilised.
In the first place, the records confirm the impression that strong winds are frequent. During thirteen years, an average of 261 gales a year with wind velocities exceeding 50 miles an hour was recorded at the summit of the mountain. This large number should be compared with the conditions at sea-level, when, even on the exposed western seaboard, few places average annually more than forty winds of such a velocity. The comparison between montane and lowland conditions may, however, be made in another form. A more recent estimate of wind-speeds has been made on Crossfell (2,930 ft.), a much lower summit in the Northern Pennines. There, it was estimated that the average wind velocity was at least twice that prevailing in the adjacent lowlands, a result comparable to similar estimates on Ben Nevis.
The Ben Nevis records also serve to illustrate the cloudiness of the mountain sky, for during the years of observation the summit was clear of mist and cloud for less than 30 per cent of the time and, as the table shows, had correspondingly low figures for exposure to sunshine (Table 2). These are, however, only different aspects of a more fundamental feature, the great humidity of the atmosphere. The average relative humidity of the air on Ben Nevis was 94 per cent of saturation with water vapour, showing little variation throughout the year, except in June, when it fell temporarily to 90 per cent, still an exceptionally high average figure.
As might be expected, this high atmospheric humidity was associated with high rainfall. Over a long period this averages 161 in annually at the summit, and it was rather higher during the thirteen years of comparative observations given in Table 2. The maximum recorded was 242 in. in 1909, and as much falls on Ben Nevis during the three “dry” months, April, May and June, as would represent the whole annual rainfall in Eastern England. High rainfall is, of course, a general feature of British mountains. Thus there is the well-known example of the Seathwaite District in Cumberland where Stye Head Tarn, east of Great Gable (2,900 ft.) has an annual average of 153 inches with a recorded maximum of 250 inches in 1928. The computed average for Glas Llyn (2,500 ft.), 500 yards north-east of the summit of the Snowdon ridge, is 198 inches. The Snowdon summit, Y-Wyddfa, in fact, competes with the head of Glen Garry (in Western Inverness), east of Sgurr na Ciche (3,140 ft.), for the distinction of being the wettest place in the British Isles. Both are considered to have an average annual rainfall of some 200 inches. Ben Nevis or Scafell and its Pike, have more of the character of isolated peaks, so that the prevalent winds can slip around them and less rain results.
The last feature of the Ben Nevis records to which attention must be directed is the range of temperatures, also given in Table 2, where they are compared with those at Fort William (at the base of the mountain).
In this table, the figures given at the foot of the columns for the year are averages in the case of temperature, and annual totals for hours of sunshine and rainfall. As there are many summits between 2,000 and 2,900 ft. to the south and west of Fort William, the rainfall there is already much higher than it would be on the outermost seacoast, and sunshine records are accordingly lower, so that the contrast between the lowland and montane conditions is much diminished.
Table 2 METEOROLOGICAL DATA OVER THE SAME 13-YEAR PERIOD
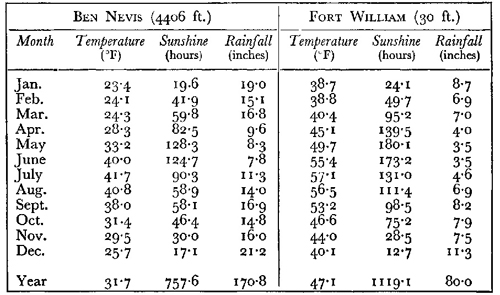
The temperature figures given in the table are for mean monthly temperatures and they bring out very clearly the striking difference in temperature conditions which higher altitude entails. At the summit, the mean monthly temperatures are at or well below the freezing point of water for eight months in the year. Even during the four “summer” months, June to September, the mean monthly temperatures barely rise above those experienced during winter at the foot of the mountain. The temperature conditions are therefore severe.
It may justifiably be urged that this represents the extreme case among British mountains and that we need a more general method of representing the usual effects of temperature. Roughly speaking, an increase of altitude of 300 ft. entails a fall in the mean temperatures of about 1° F. Assuming now that 2,000 ft. represents an approximate lower limit to the mountain zone in Britain, we can obtain representative temperatures at this altitude by taking the average of the Ben Nevis and the Fort William temperatures and adding 0·6° F. to reduce the values approximately to those at 2,000 ft. The results are included in Fig. 10.
It is interesting to note, however, that essentially similar results can be obtained for different parts of the British uplands using the varying records of temperatures made at various altitudes and calculating from them the probable values at 2,000 ft. The following table summarises the mean temperatures so obtained for January and July:
Table 3
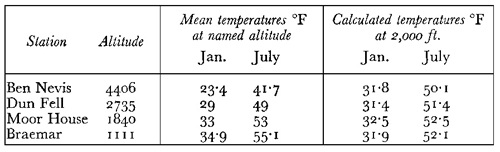
The Dun Fell and Moor House stations are two set up in the Northern Pennines by Prof. Gordon Manley, for which the data are less complete, though it will be seen that they suggest that the temperature conditions are essentially similar to those at Braemar, which represents the Eastern Scottish Highlands. The conditions at 2,000 ft. are generally similar therefore, with lower summer temperatures in the west. They may perhaps be regarded as sub-Arctic, resembling those just above sea-level in South Iceland.
The graphs in Figure 10 thus serve to illustrate what are for practical purposes the upper and lower limits of temperature for the British mountain climate. In effect, the increases in altitude produce little relative change in the levels of summer and winter temperatures but they sink, as it were, the whole temperature curve, in relation to any temperature level which may be chosen. Such a level, for example, is that represented in the graph by the horizontal line at 42° F. This level is given, because it is a temperature level which has been used
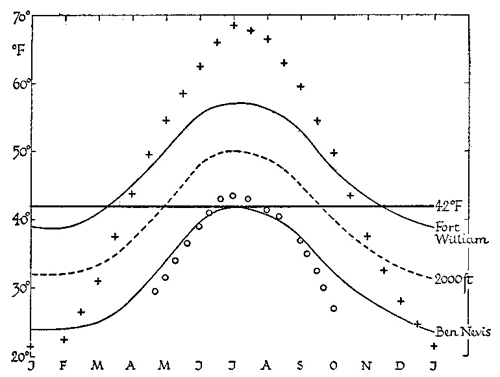
FIG. 10.—Mean monthly temperature in °F. at Fort William and at the summit of Ben Nevis (4,406 ft.)—continuous lines. The broken line gives calculated figures at 2,000 feet. The circles are summer temperatures in West Greenland and the crosses are data for Vermont (U.S.A.).
by meteorologists to represent the mean temperature above which the normal crop-plants of cool temperate climates start to grow. While the choice of such a level is somewhat arbitrary and does not by any means deal satisfactorily with the physiological problems involved, it is convenient to use this convention for the purpose of making comparisons. We could thus estimate that for plants of the type named above, the growing season at Fort William would be about eight months, say 243 days, while at 2000 ft. it would be about 142 days, and at the summit of Ben Nevis it would be quite negligible.
One of the difficulties of using such a simple method of treatment is that the higher summer temperatures at low altitudes have also a strong and cumulative effect on the rate of plant growth, as indeed do other features of the temperature cycle, such as freedom from frost. Thus lower summer temperatures markedly reduce the intensity of growth and hence the total annual amount is also very greatly affected. The strong westerly winds on British mountains tend to regulate the temperature and in particular they help to maintain lower summer temperatures than obtain on continental mountains like the Alps. Prof. Gordon Manley has drawn attention to another difference associated with the temperature curves. In spite of their moderate size, British mountains become treeless at comparatively low levels, usually below 2,000 ft., and in the same way the zone up to which useful cultivation can extend is comparatively low, often less than 1000 ft. While this is partly due to the operation of other climatic factors, it is also associated with the nature of the annual temperature cycle. If we were to go to some place such as New England, where the mean annual temperature in the lowlands is of the same order as that in Northern Britain, about 46° to 47° F., it would be found that on the mountains, e.g. on Mount Washington, the treeless zone would not be reached below altitudes of some 5000 ft. Although in Switzerland the mean annual temperatures are more widely different from our own, a similarly high timber-line is to be found in the Alps. Prof. Manley points out that this feature can be associated with the temperature conditions, for the average July temperature on Dun Fell (2,735 ft.) in Northern England is almost the same, about 48° F., as that on Mount Washington in New England at 6,284 ft.
A biological explanation of this difference is seen in the form of the temperature curves, and to illustrate the fact an additional temperature curve is given in Fig. 10. This is a typical curve for the lowlands of New England (Vermont) taken from Prof. Manley’s paper. The effect of adjusting this for changes in altitude would be to lower it to an appropriate extent. To give the equivalent curve for a height of 4406 ft., that of Ben Nevis, would require a reduction throughout of 14·7° F. Even if this were done, a large part of the annual temperature cycle would remain above 42° F. There would be a growing season at this altitude of at least 60 days and a mean July temperature of 53·8° F. Thus a considerable amount of plant growth, even from crop-plants, would be possible under New England conditions, while none could be expected with the temperature cycles obtaining in the Western Highlands of Scotland.
This method of considering the matter emphasises the importance of the low summer temperatures in British mountains as an obstacle to plant growth and as a feature which distinguishes them from localities of comparable altitude in continental areas either in North America or in Europe. In fact if we wish to find a climate equivalent in summer to that of our high mountain zone in temperature and in humidity, we must go to places in Arctic regions, preferably to those near the sea and remarkable for their frequent summer fogs, like West Greenland. But even these only rarely attain the constantly high air humidity which was observed on Ben Nevis. This, it is true, probably represents the extreme in Britain, though, judging from the rainfall records, it must be closely paralleled on the other main mountain masses in the west. Farther east and notably in the Cairngorms, where rainfall is less, air humidity is probably more variable and hence more like the Arctic stations of which we have record.
It is rather striking that the low summer temperatures found on British mountains are not associated with the presence of permanent snow, although on the highest peaks drifts may persist throughout the summer on north-facing slopes and in deep gullies. Two such drifts are well known and almost permanent, one on the north face of Ben Nevis and the other in the great corrie of Braeriach (4,246 ft.) in the Cairngorms. The latter, after having been known for some fifty years, finally disappeared for a time in the summer of 1935.
On Ben Nevis the top is usually free from accumulated snow for about 75 days in the year, though some snow may fall on about one day in ten, even in July and August. Thus, though even the highest summits are below the permanent snow-line, they are evidently very near to it. In these circumstances it might be expected that the extent and duration of “snow-lie” in early summer would have a good deal of influence on the distribution of living organisms in the highest montane zone. No detailed study of this matter has, however, yet been made in Britain.
Just as the temperature conditions differ very greatly in Britain and in the Alps, so there is also a considerable difference in other conditions. Speaking generally, British uplands lie wholly within the range of altitudes in which rainfall rises as the height increases. At higher altitudes, however (above about 5,000 ft. in these latitudes), rainfall would diminish with further increases in elevation, and this is the condition obtaining in the Alps and Pyrenees. Further, the lower layers of air are denser as well as more humid. Hence they absorb light strongly, and so higher altitudes receive much larger proportions of the sun’s energy, particularly of the ultra-violet and blue rays.
Thus Alpine conditions imply not only lower precipitation of rain or snow, but also a clearer atmosphere and intense insolation. The average summer temperatures and the illumination are much higher in the Alps, but they are accompanied by the possibility of strong radiation at night and by the certainty of great diurnal and seasonal variations of temperature, in great contrast to the small variations of temperature observed on British mountains. It is evidently better to distinguish upland climatic conditions in Britain as montane rather than alpine, and, as we have already noted, there is a great similarity between these montane conditions in summer and the corresponding features of Arctic coastal regions.
RAINFALL
The influence of the high humidity that is characteristic of British hills is not easily assessed. Atmospheric humidity undoubtedly has a considerable direct effect on plant and animal life, so that most biologists would be able to point to facts of distribution, such as the greater abundance of mosses and lichens in the western hills, which can reasonably be attributed to greater air humidity. But climatic humidity expresses itself not only through its direct effects on the distribution of living organisms but indirectly by affecting the character of the soil, and in the British Isles these indirect effects are extremely important. The only climatic data available for examining them on a sufficiently extensive scale are the rainfall data, and these we must now consider to see how far it is possible to use them in defining climatic limits. In doing this it will be necessary to adopt the following rather rough method of analysing climatic effects.
In the southern part of the Pennines, and probably generally among their eastern foothills, the average annual loss of water by evaporation is equivalent to a rainfall of about 18 in. This figure has been obtained partly as the estimate of the average amount of water lost by evaporation from a 6–ft. Standard tank, and the figures given in Table 4 are actually monthly estimates of the average losses so obtained (as inches of rain). But a similar annual figure (about 18 in.) can be obtained by comparing the rainfall over a given river basin with the “run-off” down the river, and access to much unpublished data has shown that this figure is fairly representative for the eastern Pennines. The difference between rainfall and run-off (assuming no loss into the ground or taking an average over many years) gives the net amount lost by evaporation, and we shall assume it to be distributed seasonally as in the figures given. It may be noted in passing that the problem of estimating evaporation losses may be considerably more complex than this. Empirical formulae have been worked out for estimating these losses in which it is usually assumed that they increase with increasing rainfall as well as with rising temperature.
Table 4 gives, in addition to the monthly figures for evaporation, the average monthly rainfall, also in inches, for two adjacent stations. One of them, Doncaster, lying in the Plain of York and at an altitude of 25 ft., represents a typical lowland station in Eastern England, with an average rainfall of about 25 in. per annum. The other, Woodhead, lies among the high Pennines and is surrounded by “cotton-grass” moors. It therefore represents fairly well the climate of these high moorlands, with an annual average rainfall of about 50 in. The actual figure on the hills is probably more, rather than less, than this, say 55 in.
Table 4 MONTHLY EVAPORATION AND RAINFALL IN INCHES AT DONCASTER AND WOODHEAD
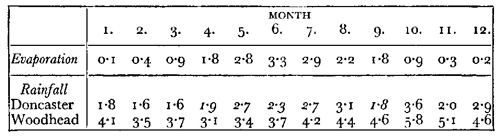
The figures show very plainly that there is no month in the year when the average rainfall at Woodhead does not exceed the evaporation. In contrast, at Doncaster, there are five months when evaporation approximately equals or exceeds rainfall (the rainfall figures in Table 4 are italicised when this is the case). Consider the implication of these facts, and particularly their effects on soil conditions. During the summer, at a station like Doncaster, the soil gradually dries out. This means that the water in the soil interspaces is replaced by air. The drains cease to run until the autumn, when rainfall once more exceeds evaporation and the water-level begins to rise in the soil.
At a station like Woodhead, on the other hand, the same filling of the soil interspaces will take place in winter, but the soils will have no opportunity of recovering and of drying out in summer, for any evaporation will be balanced by the higher rainfall. It follows, therefore, that as a whole, soils will usually be waterlogged in a rainfall of the Woodhead type and only those on considerable slopes will have a chance of becoming drained and well aerated. We may thus recognise that in a rainfall of this type and magnitude there will be a strong tendency towards bog-formation, and it may perhaps be useful to note that in Britain a rainfall of 50 to 55 in. (that is, about three times the evaporation figures) will apparently suffice to give conditions favourable to bog-formation. This is a useful measure, even if a rough one, of the effective humidity of an upland climate.
The influence of high rainfall is exerted in another manner also. When rain falls on soil and percolates through it, the water naturally carries away in solution and into the drainage system any soluble mineral salts present in the soil. These will include most of the substances valuable as plant food as well as the lime which prevents a soil from becoming sour. The process is called leaching, and the rate of leaching will obviously depend very largely on the rainfall. When this only just equals the evaporation losses there will be little or no leaching, but the higher the rainfall becomes in comparison with evaporation, the more rapid leaching will be. Very roughly, then, we shall expect little or no leaching when the rainfall is about 18 in. per annum, but where the annual rainfall is 54 in. we may expect leaching to proceed at about twice the rate expected under a rainfall of 36 in. It will be realised that these rough comparisons as to leaching apply only to porous soils through which water can freely percolate and there is obviously no need to stress the numerical comparison, although it serves to emphasise the high rate of leaching found in upland areas, where a rainfall exceeding 54 in. per annum is common.
The analysis carried out in the preceding paragraphs gives us one method of obtaining a significant boundary of humidity which must have pronounced biological effects. It is perhaps worth noting that a similar figure, an annual rainfall of about 55 in., has been obtained by noting the rainfall at upland sites where reclamation of moorland has proved just possible or has failed. If allowance is made for the nature and porosity of the underlying rock, this is roughly the altitude at which habitation ceases, and in the northern Pennines and eastern Cumberland it is stated to lie very near to the point at which rainfall exceeds 55 in. Of course, this is an extremely indirect method of approaching such a problem, for the result must be greatly affected by the nature of the prevailing occupations in the district examined; nevertheless in this particular instance the relation is clearly one which operates through soil effects, so that it agrees with the conclusion already reached in suggesting that the rainfall indicated is one of distinct biological significance.