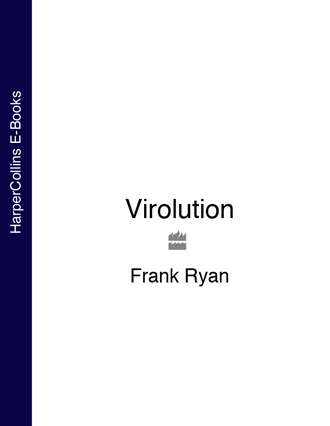
Полная версия
Virolution
The medical approach includes prevention, through genetic counselling, public education about the risks of increasing maternal age, avoidance of risk factors such as radiation of the germ cells and foetus, caution over drug and chemical exposure, such as thalidomide, and vaccination against the rubella virus, which is known to damage the developing foetus. Newer genetic measures, such as in vitro fertilisation of the sperm and egg, followed by genetic screening of the resultant foetus when it is at the stage of a ball of cells, can be offered to high-risk families. Known as pre-implantation genetic diagnosis, or PGD, this may be helpful in a variety of diseases, including sex-linked disorders, single gene defects and chromosomal disorders. The potentially amenable sex-linked disorders include haemophilia, fragile X syndrome, most of the neuromuscular disorders (currently there are more than 900 recognised neuromuscular dystrophies) and hundreds of other diseases. Indeed, the potentially amenable single gene defects also include cystic fibrosis, Tay-Sachs disease, sickle-cell anaemia and Huntington’s disease.
As a general rule, we can see that a genetic abnormality is more likely to respond to PGD if it is predictable, because the genetic inheritance is known, and if its effects can be demonstrated in isolated embryological cells. Some people will have ethical objections to such manipulations of the human embryo, but for governments and the groups who monitor the ethics of medicine, the advantages to families will usually outweigh the ethical worries. It is also important to grasp that pre-implantation genetic diagnosis, with selection for healthy embryos, not only removes the risk of serious disease in an affected offspring but in some cases also eliminates the risk to future generations of the family.
A key development over the last decade or so has been our increasing understanding of the role of mutation in cancer.
But before we enter this intriguing, and disturbing, domain, we should spend a minute or two addressing some key questions as to the essential nature of what we are dealing with. What is cancer? Where does it come from? And why does it frighten us so much?
Cancer is a term used for diseases in which our own body’s cells divide without control and are able to invade other tissues. To put it another way, cells that have been programmed to work in perfect harmony with all the other cells, tissues and organs of the body, go ape and declare violent independence. What is at stake, for the aberrant cells, is immortality. Indeed, cancer cells are immortal in cell culture – but such ambitions are disastrous for the tissue, organ, and individual in which such ambitions arise, since it means that they invade the local tissue, or organ, and from there invade the local environment, or bloodstream, where they cause havoc, and possibly the death of the individual.
There are more than a hundred different forms of cancer, often named after the organ where they occur, such as the colon or breast, or after the kind of body cells they arise from, such as “carcinoma”, which arises from skin, or the cells lining internal organs, “sarcoma”, which arise from internal tissues, such as bone or muscle, “leukaemias”, which arise from blood-forming cells, and “lymphomas” and “myelomas”, which arise from cells involved in the immune system. In the words of Professor Karol Sikora, former chief of the WHO cancer programme, ‘Cancer is frightening because it is the enemy within.’4 It is also frightening because it is common. One in three of us in developed countries will develop cancer at some point in our lives. In 2008, in the USA alone, some 1,437,180 people were newly diagnosed with cancer, and that same year, in the UK, 1.2 million people were living with the disease from day to day. One of the ironies is that with improvements in healthcare as a result of modern treatments, the numbers of people living with the disease are likely to rise, with Sikora estimating a rise to 3 million in the UK by the year 2020. Indeed, it seems that never a day goes by without a cancer story in the news, with sufferers or their loved ones describing their experiences, and tribulations, on television, in newspaper and magazine articles, or on the personal pages on the Internet. Indeed, if we Google for cancer we discover approximately 300 million websites worldwide. Even the medical term for it and the defining words are hardly reassuring: “a malignant neoplasm”, a disease in which the body’s own cells display “uncontrolled growth”, followed by “metastasis”, which means the invasion of other organs of the body.
We all know that cancer is one of the common diseases and a significant cause of death in any country. We also know that cancer tends to get commoner with increasing age. Many of us probably also know that the term “cancer” is derived from the Latin word for a crab, which would appear to imply that it is a creeping thing that, like the splay of the crab’s many legs, spreads and invades our tissues. In fact, let me assure readers that many cancers are eminently treatable, far more so than when I first qualified as a doctor, and some are even completely curable. As with anything that frightens us, it becomes a good deal less frightening when we come to understand it better. And there can be no doubt at all that the logical approach to cancer, and its treatment, comes from exactly that – from understanding.
Our body is composed of organs and tissues, such as the brain, heart, and the glandular tissues that line the breast, or the prostate, and these in turn are made up of many different types of cells. As part of the wear and tear of life, cells die and must be replaced by the division of neighbouring cells. The first step in understanding cancers is to grasp the fact that nearly all cancers are caused by disturbance in the way genes, and other regulatory factors, exert control over this pattern of reproduction of cells.
Two groups of genes appear to be particularly important in controlling the way cells reproduce themselves. One group, known as “oncogenes” (onco here means tumour), are so-called because if they are inappropriately activated they increase the risk of developing a cancer. A second group are known as “tumour suppressor genes”. As the name suggests, these normally suppress the tendency towards uncontrolled cell proliferation that is such a prominent feature of cancers. Mutations that inappropriately switch on oncogenes or inappropriately switch off tumour suppressor genes are thus a potent cause of cancer. The decoding of the human genome has highlighted the genetic alterations that underlie cancers in such unprecedented detail that it has led two American oncologists, Vogelstein and Kinzler, to declare that ‘cancer is, in essence, a genetic disease’.5 They have summarised the mutated genes responsible for various cancers, together with the ways in which these mutations have perverted the normal genetic mechanisms to do so. For example, one in five familial breast cancers have been linked to mutations in the genes BRCA1 and BRCA2. Geneticists can further predict that women who carry these mutations have an 80% risk of developing breast cancer during their lifetime, so that pre-emptive surgery offers the potential of prevention. Recently, PGD has also been extended to help such families, and embryological screening has been made increasingly available for BRCA1 and BRCA2, with the first assisted babies, freed from the terrible risk, already born in a number of countries.
In 2006, a multi-centre screening programme in the USA looked at more than 13,000 genes taken from human breast and colon cancer cells, enabling authorities to compare the genes they found in the two cancers with the normal, and revealing that individual tumours accumulate an average of 90 mutant genes.6 Meanwhile, they concluded that a much smaller number of mutations are critical to the early stages of the cancer process, in their estimation perhaps 11 mutations for each of breast and colon cancer. Encouraged by these findings, the US National Institutes of Health is drawing up an atlas of cancer genomes – the Cancer Genome Atlas, or TCGA – with the aim of decoding the genomes of every human cancer and, by comparing these to the normal, extrapolating the genetic abnormalities that underlie all cancers.7 A pilot study has begun with cancers of the lung, brain and ovary.
It is not unreasonable to anticipate, as our knowledge of mutation grows, that important preventive and therapeutic aspects will come from it. However, though the understanding and medical applications of mutation have proved to be helpful, mutation is neither the exclusive mechanism of hereditary change in evolution nor the exclusive explanation of the genetic underpinning of disease, including cancer.
3
The Genetic Web of Life 1
Sit down before fact as a little child, be prepared to give up every preconceived notion, follow humbly wherever and to whatever abysses nature leads, or you shall learn nothing.
THOMAS HENRY HUXLEY2
When, on a hot afternoon in September 1994, I arrived at the Rockefeller University, New York, with an appointment to interview its distinguished president, and Nobel Laureate, Joshua Lederberg, I considered myself fortunate that he had agreed to see me, since he was one of the busiest men I was ever likely to meet. The meeting with Terry Yates, two months earlier, had radically altered my perspective on viruses, and, on my return to England, I had consumed what literature I could lay my hands on concerning what for me was a new topic of inspiration – the possibility that what we were observing in pandemic plagues, including AIDS, might best be interpreted as evolutionary phenomena. I had arrived early so I took a walk down York Avenue to 68th Street, turning towards the river by the twin-fronted colossus of the New York Hospital, until I reached a low concrete parapet on which I could lean and gaze out over the wide East River, with its turbid, black-green water.
I had been here once before, while working on my book on tuberculosis, and the sight of the hospital brought back poignant memories. Rene Dubos, a scientist I greatly admired, had worked at the Rockefeller University for most of his life. A scientist-philosopher, and twice a Pulitzer Prize winner for his writing, Dubos was one of the most original thinkers among the scientists involved in the antibiotic story. He had pioneered the discovery of the soil-derived antibiotics, such as streptomycin and neomycin, and had played an important part in the discovery of the cure for tuberculosis. I knew that it was my writing about Dubos in my book on tuberculosis that had opened Lederberg’s door to my interviewing him. But Dubos’s contribution to the discovery of antibiotics, and the cure for tuberculosis, had ended abruptly, and tragically, right here, in the New York Hospital, where his first wife, Marie Louise, had died from the disease. I couldn’t help reflecting now on Dubos, and his highly original way of thinking about microbes, including viruses, as I gazed upriver towards the looming ironwork of the Queens-boro Bridge. Viruses appeared to be omnipresent. In fact, whenever we bothered to probe any life form on Earth for the presence of viruses, we seemed to find them. It made little sense that at this time only some 5,000 strains, or species equivalents, of viruses were known. Only recently had we discovered that viruses teemed in the oceans, where we had little or no knowledge of what they were doing – yet the vast numbers alone suggested that their presence was significant. We knew, by now, that most, if not all, life forms had viruses that invaded them, and, given that there were millions of different species inhabiting the Earth, it was clear that our knowledge of viruses, even at this very basic level, was inadequate. Those two months of intense background reading and research had convinced me of this. It had also convinced me that, in our blinkered vision of viruses, we were missing something very important. These questions troubled me as I stood in Founders’ Hall, pausing in the reception area before a painting of its first medical director, Simon Flexner, who had earned a distinction that perhaps only a doctor would appreciate – of having the dysentery bacterium, Shigella flexneri, named after him. I climbed into a battered green-and-black elevator old enough to have been familiar to Flexner, and I widened my stance, a trifle warily, as it rattled and groaned on its way to the fourth floor.
I shook hands with Lederberg in a room cluttered with boxes of scientific papers and lantern slides, its walls be-decked with a proliferation of certificates and diplomas. He sat down opposite me, bald-headed and stolid as a Buddha. ‘Well,’ he remarked, his eyes following my gaze with a slight twinkle, ‘they are rather an idiosyncratic collection … I got to microbiology through genetics – through biochemical genetics in particular. My first experience in that area was with your namesake, Francis Ryan, who was my mentor at Columbia University. You don’t have Joseph after your first name?’
I shook my head.
‘A wonderful man. He was the first post-doc to join Beadle and Tatum in their laboratory at Stanford at the very beginnings of biochemical genetics. He was working on mutations leading to nutritional deficiency in Neurospora. I entered Columbia College in 1941. Francis was away that year, but Beadle and Tatum’s paper had just been published and I knew he was there. I just waited for him to come back and pounced on him in his laboratory.’
We had already taken our seats, among the piles of journals and papers as I inched the line of conversation along. ‘But there must have been something even before that that made you go to college with this interest?’
‘Well, that’s a somewhat broader canvas. I can’t give you the ultimate answers to that question. But from the very beginnings of my recollection, from when I was about five years old, I recall that I was devoted to science. I had no doubt I was going into science, probably medical science, so I prepared myself for it.’
‘Was there a history of science in your family?’
‘Not at all. My father was an Orthodox rabbi. I don’t think there was a total disconnection between his vocation and mine, but there was a generational polarity.’
I paused to consider this curious phrase. ‘Perhaps what you had in common was a certain preparedness to discuss life, and perhaps a philosophical attitude of mind might have contributed?’
‘Oh, I think so. Issues of learning, of enquiring … A life in discovery was compatible with my secularism.’
‘Can I ask another question? How old were you when you were awarded the Nobel Prize?’
‘I was 33. They took their time about the award. I was 21 when I did the work.’
Of course, I already knew that the youthful Lederberg began medical studies at Columbia’s College of Physicians and Surgeons, but even then he was inspired by Oswald Avery’s work at the Rockefeller University, which had led Avery to propose that DNA, and not the widely assumed protein, was the gold dust of heredity. This had been critical to Watson and Crick’s later discovery of the chemical structure of DNA, which transformed genetics and our understanding of evolutionary biology. And Joshua Lederberg had played his part in this fabulous story.
Even as a student, he had refused to believe the widely held opinion that bacteria only made identical genetic copies of themselves. It was why he had written to Edward Tatum, Ryan’s postdoctoral mentor at Yale University, asking if he could come and work with them. The first publication to come out of this collaboration was in the names of Lederberg and Tatum and covered less than half a page in the letters columns of the journal Nature, on 19 October 1946. It carried the title “Gene Recombination in Escherichia coli” – E coli being a common bowel bacterium – and it proved, for the first time, that bacteria can pass on genetic information from one strain to another, a process we now call “bacterial conjugation”.3 In this sense the word “conjugation” is from the same stem as our human term for the “conjugal” rights of marriage. Indeed, at the close of the paper, Tatum and Lederberg had made it perfectly explicit that “These experiments imply the occurrence of a sexual process in the bacterium.”
The piquant truth is that Joshua Lederberg was awarded the Nobel Prize for the discovery of sexual relationships between bacteria.
Sex is a perfectly normal, evolved, behaviour, which is found, sometimes accompanied by beguiling mating rituals, in virtually all animals as well as plants and simpler life forms. The fact that bacteria use a sexual process to swap genetic information is important to medicine, explaining some instances of bacterial resistance to antibiotics. And this topic afforded a perfect springboard for the deeper explorations of our conversation, which lasted most of the afternoon. I was fascinated, in particular, by his long-held view that ‘terrestrial life is a dense web of genetic inter-reactions’. I was keen to hear more of what he meant by the expression.
Perhaps, he suggested, we should look at living organisms as metabolic nets, capable of reaching out and accepting help, at chemical or even genetic level, from other life forms. ‘On the one hand, each life form is coded by its own genetic make-up, but there is an interdependence there. We can’t survive without taking advantage of the genetic machinery of plants.’ Of course, he was referring to photosynthesis, which enables plants to make sugars and amino acids that animals, such as we humans, rely on for life. ‘So, in this sense,’ he insisted, ‘we are symbiotic with plant genes.’
I was interested in his evocation of the concept of symbiosis. It reminded me of the fact that he had referred to symbiosis again and again, in the headings and subject matter of his book chapters and scientific publications. This suggested that he had given careful thought to its role in life.4
‘There are,’ he explained, ‘marine invertebrate animals that have carried this further, so that instead of bothering to eat plants they embrace algae living inside their skin. Many of the well-known bacterial symbioses with insects are not so fundamentally different from that. In these cases there is an integration of genetic machinery, even though the interacting genomes are still distinct. The symbionts are in different cells, and they could be parted asunder. But I see a continuum between that phenomenon and the kinds of symbiosis where the two organisms occupy the same cell, such as we see in plants with their chloroplasts. It’s not so difficult to extrapolate from that to the evolution of invertebrates, where you have algae living in the epidermal cells. But what we find in the chloroplast has taken the concept further. The primordial chloroplast has itself exchanged considerable numbers of genes with the nucleus. Meanwhile, some genes that were undoubtedly nuclear have found their way into the chloroplasts. So these have not been pure genomes for many aeons.’
I suggested that these ideas would surprise many biologists, and geneticists, who were fixated on the idea of genes being handed down in a simple, vertical, way from parents to offspring.
‘You just need some scaffold to begin your thinking. Then the more you learn the more you realise that the exceptions are almost the rule.’
I was eager to extrapolate this line of reasoning to what really interested me at this stage. ‘The popular conception of a virus is something necessarily nasty, something that infects people and makes them ill – sometimes kills them. But can you conceive that viruses in nature might also have a symbiotic role with animals?’
I was well aware in asking this question that, as early as 1952, Lederberg had published a landmark paper under the title, “Cell genetics and hereditary symbiosis”.5 In this paper he had proposed a new scientific term, the “plasmid”, to cover all sorts of hereditary packages that crossed the genetic divide between different life forms. In this same paper, he stated outright that plasmids were symbiotic organisms that formed part of the genetic inheritance of the life form to which they contributed this new genetic information. From my perspective, this transfer of pre-evolved genetic information was quite different, from an evolutionary perspective, to the Darwinian concept of random changes in the coding sequences of genes arising through errors in copying DNA when cells divided.
He said: ‘It’s a very interesting question.’
We talked about how viruses could change the behaviour and internal chemistry of bacteria, for example by making them resistant to antibiotics. The diphtheria bacterium produced a poison, known as a toxin, which was entirely dependent on the presence of a virus within the bacterium.
So it was that our conversation moved round a topic that we both recognised as extremely important, if potentially very controversial.
I explained what I had learnt from the scientists investigating the hantavirus epidemic, for example the fact that baby deer mice are born without the virus. They acquired it as weanlings, from copious secretions of the virus in the urine and other excreta of the mother. Yet when they acquired this virus, which was so horribly lethal to people, they showed no sign of illness. It was as if, in first meeting the virus when their immune systems were just coming to recognise self from alien, they came to regard the virus as self. In fact, some of the biologists working on the virus-mouse interaction had the feeling that the baby mice grew bigger, stronger, as a result of the presence of the virus. I took a breath and asked the question that had preoccupied my thoughts for the last two months.
‘I know that viruses don’t think. They don’t have a concept of good or bad – they’re not just immoral but amoral. But is it possible a virus could have a beneficial effect on an animal species?’ I should have known better than to use the word “beneficial”, since it is loaded with anthropomorphic overtones. What I meant, and should have asked, was if the presence of a virus might help the host survive.
‘Well, that would be interesting … I don’t know of a clear example of any such mutualistic advantage, but it’s on the cards. And if nothing else, cross-immunity to other infecting agents is certainly going to come into the picture. But I just don’t happen to have it at my fingertips for animals.’
I pushed it a little further. ‘I find myself asking the question, could a viral infection in a species change that species – could it go so far as to create a new species?’
It was probably the most challenging question I put to him, and it resulted in another of those telling pauses.
‘I can commend a book to you that has just come out. It answers the somewhat larger questions. It is by Jan Sapp and it covers symbiosis – the history of the concept.6 Jan is a historian of science from York University, in Canada. He was a visiting scholar here in my laboratory when he wrote the book. He’s been following the thinking of Lynn Margulis, who is probably the most articulate person on this line of thinking. You might have seen something of her writings. Where symbiosis leads to the convergence of two genomes from disparate sources, making, if you like, a very wide hybrid, it becomes the source of evolutionary change of the most major implications. There is a fair consensus now that this is how the eukaryotic cell evolved.’
The eukaryotic cell is a cell with a nucleus. The evolution of such a cell from humble bacterial forebears gave rise to all of the animals, plants, fungi, algae and smaller creatures, such as the amoebae of my school biology days. That same evolutionary step had been extolled by the eminent Darwinian, Ernst Mayr, as the single most important step in the evolution of life. If my interview with Terry Yates had first opened my eyes to the possibility of a new vision of viruses and their role in evolution, this interview with Joshua Lederberg had further encouraged that vision. I left New York more determined than ever to examine it further.
In the opening chapter I outlined a three-way symbiotic relationship between the sea slug Elysia chlorotica, its host alga, and an unknown, virus, putatively a retrovirus, that has entered into a persistent relationship with the slug. But back in 1994 I knew nothing about Elysia, and its relationship with the virus was poorly understood. The truth is that I was in the dark as far as symbiosis was concerned. I had no idea how this biological condition called symbiosis was defined. Did symbiosis imply a different evolutionary mechanism from the highly respected modern Darwinism? My conversation with Lederberg suggested that there were important differences between the two evolutionary disciplines, yet there was no hint that he felt these differences negated the conventional viewpoint. I was mindful of his words of advice: ‘You just need some scaffold to begin your thinking.’ My scaffold would be the biological discipline of symbiosis, and its many examples and operative mechanisms, focusing in particular on how symbiologists – the people who study symbiosis – figured that symbiosis operated as an evolutionary force.