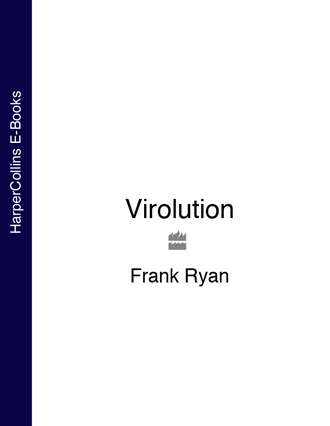
Полная версия
Virolution
In such tragic circumstance arrived a new or “emerging” plague into the Four Corners States of New Mexico, Arizona, Utah and Colorado. Although it began in the territory of the Navajo Nation, it soon manifested in the non-Navajo areas of the other states, and spread far and wide throughout the rest of America. But the epicentre was always focused on the desert areas of New Mexico and Arizona, where it terrified the local community, often infecting previously fit young people who could be reduced from rude good health to death, with whited-out lungs, in 24 hours. When I first arrived in New Mexico, the plague was still rampant, with the entire intensive care unit at the University Hospital in Albuquerque devoted to looking after newly diagnosed cases. Through intensive modern investigation and devoted medical management by the medical staff in various cities and hospitals, the death rate had been clawed back from an initial 70% to something closer to 50%. My purpose in coming to New Mexico was to examine new, or “emerging plagues”, and particularly those caused by viruses, such as Ebola and HIV-1, as part of the researches for my book, Virus X,5 and so the emergence of this all-American plague afforded me a rare opportunity to examine the most intensive modern medical and scientific response, in day-to-day detail. I found that the investigation of the plague, and the medical management of its victims, was still at its height, and I was duly grateful to my hard-pressed and dedicated colleagues, who allowed me to sit in with them in their clinical meetings and scientific experiments.
I knew that scientists working in the Special Pathogens’ Branch of the Centers for Disease Control in Atlanta – the world-famous plague hunters – had discovered that the Four-Corners’ epidemic was caused by a hitherto unknown virus. Here then was the opportunity to see for myself where such emerging viruses came from in nature, and why they behaved as aggressively as they so often did when they encountered a new host, such as our human species. In Albuquerque I would spend some time observing the doctors in the University Hospital, fighting to save the lives of infected patients. Here they did me the great courtesy of allowing me to interview victims and their relatives, with the normal medical confidentiality, and to witness for myself the harrowing experience of contracting an emerging plague virus. I travelled to Atlanta to observe the work of the virologists and geneticists at the Centers for Disease Control, where they had first realised that they were dealing with a newly emerging virus, which was a member of the genus of viruses known as the “hantaviruses”, using molecular techniques only 13 days after the onset of the epidemic. It took them six more months to see the actual virus, which would subsequently be called the Sin Nombre hantavirus – the hantavirus with no name. I sat in on the online discussions between the virologists and the epidemiologists at CDC and the internists and medical investigators in Albuquerque. I found myself gazing with curiosity at images of the new virus taken with the electron microscope, which looked as innocuous as minuscule balls of cotton wool. I moved on to California, where I interviewed virologists who had investigated the first African outbreak of Ebola and who had been intimately involved with the investigation of the emerging virus we now know as HIV-1, the cause of the AIDS pandemic. It was towards the end of my exploration of the Sin Nombre outbreak, and after I had collected a great many interviews with the scientists investigating it, that I returned to Albuquerque to talk toTerry Yates, one more interview as I supposed among many, when I wanted to look at what the biologists had discovered about the animal source of the virus.
I knew by then that the virus that was so lethal in people had come from the commonest rodent in America, the humble deer mouse, the equivalent of the common field mouse back in the UK. And in that conversation, I sensed the ground shift beneath my feet, as I listened with a growing astonishment to the slightly built and dark-haired Yates as he explained, with a fast and engaging Kentuckian accent, his own take on plague viruses, and hantaviruses in particular.
In addition to his chair in zoology, Yates was also the director of an important biological field reserve in New Mexico, known as the “Sevilleta”, where biologists are conducting one of the most comprehensive ecological surveillance programmes in history. This, in major part, is where the gigantic collection of mammals in the museum has come from. Yates was a world-famous expert in mammalian evolution. He did not focus, as most biologists do, on a single species or even a genus. His interest lay in evolutionary systematics: in the patterns and processes that lead to the diversity of animal species and how this gives rise to the branching tree of their evolution. The outbreak of the Sin Nombre hantavirus epidemic in his own back yard added a new, and unforeseen, practical significance to the theoretical work he and his team had been engaged in for decades. Not only could Yates and his fellow scientists look for the hantavirus in living deer mice, but they could also extract viral sequences from the vast trawl of carcasses stored in the museum and from there they could trace important facts about the virus’ own evolution. What then, I ventured to ask him, was the ultimate purpose of this exercise?
‘We’re interested,’ he told me, ‘in the co-evolutionary potential of the hantavirus as it evolves in direct parallel with the host.’
His reply surprised me. Like most doctors, I thought of viruses as nothing more than parasites. I was taught modern Darwinian evolution as part of the core biology that underpins our understanding of medicine. Lay people often confuse viruses with bacteria but they are radically different organisms. Most viruses are a lot smaller than bacteria, so small in fact that most are completely invisible even under the highest magnifications of the light microscope. Only through more subtle detective work, using immunological probes, or molecular chemistry, or, ultimately, the vast magnification of the electron microscope, can we bring them into focus. In their genetic arrangements viruses also differ markedly from bacteria. Their DNA is usually packaged in the linear clusters we call genes, rather like our own, while the DNA of bacteria is packaged in a single ring. Viruses are also the ultimate masters of evolution through mutation. They mutate with astonishing speed, something like a thousand times faster than bacteria, which in turn mutate approximately a thousand times faster than we do. Mutation of this order is an important consideration for medicine since it is one of the ways in which bacteria and viruses become resistant to antibacterial and antiviral drugs. For example, it is the key to understanding resistance to therapy in conditions such as AIDS and tuberculosis, where the phenomenal mutational capacity of the HIV-1 virus and the tuberculosis bacterium means that we are obliged to prescribe a cocktail of different drugs to control them.
In this conversation with Terry Yates, I discovered that many different species of rodents around the world appeared to have hantaviruses that infected them. The first hantavirus ever discovered came from a rodent in Korea – it emerged close to the Hantaan River, which gave its name to the genus of viruses as well as to the human illness the virus caused, which is known as Hantaan fever. So when Yates told me he was looking into the evolutionary aspects of the hantaviruses, I presumed that he was talking about mutation. I knew nothing about the co-evolution of a virus in parallel with its host. Indeed, I was still thinking along the conventional medical and evolutionary lines when I asked him another question.
‘What do you perceive as a virus?’
‘That’s a good question. Some people will argue that viruses are not life. I disagree with that, certainly. Perhaps a more pertinent question to me is not “what is a virus?” but “what is a species as far as a virus goes?” Are there species of viruses analogous to mammalian species? Basically I am only recently into viruses. I became interested, almost accidentally, because of the work we do with mammals. It just happens that mammals are the main reservoirs of these viruses. So for me the question “what is a species when it comes to viruses?” is best understood in the context, are they evolving, and are they co-evolving with their reservoir organism? I think that viruses are lineages that have their own evolutionary trajectory and their own historical fate. By analysing the history of that evolution and studying the branching patterns of those viral lineages, we can define viruses based on the branches of the tree. And when we look across the board at these hantaviruses, our evolutionary analysis thus far has shown a very tight correlation between the evolutionary tree that illustrates the history of the mammalian host and the evolutionary tree that illustrates the history of the viruses.’
This still made little sense to me in the way I had been educated to think about viruses, not from the medical perspective and not, as I was already beginning to suspect, from the evolutionary perspective.
‘When you say you are studying the virus, do you imply studying its genome?’
Here I should explain, for my non-scientific readers, that a genome is the sum total of all the genes of a life form. While all other forms of life, including humans, have genes coded by DNA, viruses can be coded by DNA or, as in the case of the hantaviruses, by its sister molecule, RNA.
‘Studying its genome,’ he confirmed, ‘or any other characters that would be applicable. They are such simple organisms that – for example, in the case of hantavirus – to get enough characters to be able to understand the evolution of the virus, the best thing to use is the RNA sequences. So we’re having better luck with this because each of the bases in the RNA sequences is applicable.’
‘How do you manage to do that? Have you been studying the viral genome as and from when you first noticed it, or have you some way of going back in time to see what the virus was like some time ago?’
‘That’s a complicated subject and one that has been debated at the Natural History Museum at the exhibit on the evolution of man. We use a methodology called Cladistics. It’s basically a phylogenetic analysis [tree of life analysis] of the different lineages of viruses.’
‘This is where you observe them in different species?’
‘Not exactly, no. We’re talking about [analysing sequences over] enormous periods of time here. We have been successful in extracting viruses from our frozen tissue collection and we are having success with extracting DNA from fossil organisms. People have successfully amplified and sequenced DNA from plants that were embedded in Miocene rocks – these are 30-million-year-old plants. So what you can do with this phylogenetic analysis is take a viral sequence from a hantavirus of the Four-Corners deer mouse and compare that to other species of viruses that occur on other branches [of the parallel virus-rodent trees]. From this you can extrapolate what the historical condition was. So we can trace the evolutionary sequence back in time and make comparisons to other lineages that diverged from the lineage you are interested in, much earlier in time.’
The implications were slowly dawning on me. ‘So you see a link between the virus and the mammal that is very close?’
‘That’s right. For example, if we were looking at eutherian mammals [the placental mammals including humans], we might compare the sequences of eutherian viruses to those of the marsupials and egg-laying mammals, which are more ancient.’
‘Because they are similar, but not the same viruses, you raise the question that sometime in the past, just as the animals had a common ancestry, perhaps their viruses might also have had their own common ancestry?’
‘That’s right.’
A surprising idea had entered my mind. From my background knowledge of evolutionary biology, and in particular of evolutionary virology, I could assume that virologists, sharing the same conventional viewpoint as I had up to now, would assume that the viruses, given that they mutate at a vastly faster rate than the mammals, would fast-track their own evolutionary trajectories, to stay close to the evolutionary pathways of their mammalian hosts. But now, thanks to the surprising idea that Terry Yates had planted in my mind, I asked myself the question:
What if both the virus and its mammalian host are influencing one another’s evolution, one evolutionary tree interacting with the other, over the vast time periods of their co-evolution?
I spent a good deal longer than I had initially envisaged with Professor Yates, visiting the Sevilleta and enjoying the courtesy of a stay with him and his family, when I had ample opportunity to examine his work, and to think about his ideas in more detail. When I put my question to Yates himself, he could provide no definite answers other than the observation that viruses and hosts appeared to be following very close co-evolutionary trajectories. Nevertheless, over the months that followed, his explanation of virus-mammalian co-evolution intrigued me deeply and it caused me to look much harder at the relationships between viruses generally and their hosts. In particular I spoke to other biologists, and especially virologists, and I explored the literature far and wide. As far as I could determine, nobody was even thinking along the lines of viruses and hosts influencing each other’s evolution. And thus it would appear that, entirely by chance, I had stumbled across an idea that, if true, would have major implications. It was one of those exciting moments a scientist hopes will come along at some stage of his or her career, a new idea that makes you think long and hard, and even to question some of those ordinary assumptions you have carried with you since your undergraduate years.
What, then, is a virus?
Biologists will differ very widely in their answers to this question. Some will quote the distinguished immunologist and writer, Sir Peter Medawar, Nobel Laureate for his work on tissue transplantation, who caricatured the virus as a piece of bad news wrapped in a protein. But this definition, however whimsical, adds little to any real understanding. Others, usually molecular biologists or geneticists, will adopt a chemical perspective, while Darwinian evolutionists – and until recently symbiologists too – are inclined to see viruses as mere agents of “horizontal gene transfer” between different species. We saw a very interesting example of this with Elysia chlorotica, when the strange retroviruses in the slug’s genome appeared to enable the transfer of key genes “horizontally” across the kingdoms of plants and animals, as represented by the alga and the slug. Another interesting perspective is that of Eckard Wimmer, a professor in the Department of Molecular Genetics and Microbiology at Stony Brook, New York, who became famous in 2002 for reconstructing the polio virus from mail-order components back in his lab.6 This experiment provoked a good deal of interest and notoriety. But what Wimmer and his co-workers wanted to do, amongst other things, was to make a conceptual, and perhaps philosophical, point. If you know the genetic formula of a virus, you can reconstruct it. They even quoted an empirical formula for the polio virus, as follows:
C332,652H492,388N98,245O131,196P7,501S2,340
It is strange to think of an organism, even if exceedingly small, being reduced to a list of atoms. One is reminded of the bitter opposition of the gentle French naturalist, Jean-Henri Fabre, the so-called poet of entomology, who, although he greatly respected Darwin as a man and fellow scientist, opposed Darwin’s line of thinking. In Chapter VIII of his book, More Hunting Wasps, Fabre described a ‘nasty and seemingly futile’ experiment he had conducted, rearing caterpillar-eating wasps on a ‘skewerful of spiders’. We need not consider the experiment in detail here, only Fabre’s conclusion, which led him to dismiss the concept of evolution through natural selection. In Fabre’s own words, ‘It is assuredly a majestic enterprise, commensurate with man’s immense ambitions, to seek to pour the universe into the mould of a formula … But … in short, I prefer to believe that the theory of evolution is powerless to explain [the wasp’s] diet.’
It is perfectly true that, in certain circumstances, viruses do behave like inert chemicals. Indeed, I once performed a series of experiments that proved this. When I was a medical student at Sheffield University, I was interested in how our mammalian immune system would respond to viral invasion. The penetration of such alien organisms into our bloodstream – literally the very heart of our being – would be a major, and extremely threatening, event. With the help of my mentor, Mike McEntegart, Professor of Microbiology, I set up an experiment in which I injected viruses into the bloodstream of rabbits. Some readers might react with concern about hurting experimental animals, but the virus I used was a bacteriophage, known as ΦX174 – a virus that only attacks bacteria – so the rabbits suffered no illness. Yet their adaptive immune system responded in exactly the way it should to any alien invader, with a build-up of antibodies in two waves, rising to a peak by 21 days, where a single drop of their serum would be seen to inactivate a billion viruses in mere moments.
The point I am making is that this experiment, by its very design, did not really reproduce the living behaviour of viruses. Injecting a virus into such a host was the virological equivalent of landing people, unprotected, on the surface of Mars. The circumstances were unnatural to the virus and it could neither survive nor respond, in the behavioural sense, and so it died. Had I injected smallpox, or influenza, or HIV-1, into people, the result would have been altogether different. The virus would have come alive in its natural host and a fearsome interaction, virus-with-human, would have followed. As this suggests, it is a waste of time, from the definitional perspective, to consider viruses outside of their natural ecology. Outside the host, it could be argued that a virus really does behave much as Professor Wimmer’s formula – as an inert assemblage of genes and proteins. Only in the real circumstances of its life cycle, when it interacts with its natural host, do we witness the real nature of viruses.
This is why, like Terry Yates, I take the view that viruses, in their natural life cycles, should be regarded as life forms. In this sense the extreme reductionism of depicting a virus as a list of chemicals is implicitly absurd. We might similarly contrive a chemical formula for a human being, when we would end up listing a similar collection of atoms, albeit their numbers would be far more gargantuan. People who view viruses only as chemical assemblages miss the vitally important point that viruses have arrived on the scene through a vast, and exceedingly complex, trajectory of evolution, much as we have ourselves. And though Professor Wimmer might seem to be promoting the viewpoint of a virus as inert, this is not his thinking at all. His view of viruses is much the same as my own, and that of the majority of biologists. A virus may appear inert outside its host, but when it enters the host cell, he too regards it as coming alive. And what an extraordinary life form it turns out to be – for here, in the landscape of the host cell, it has the unique ability of taking over and driving the host genome to make it manufacture new viruses.
Here, in the cells of their natural hosts, viruses are born, like all other life forms. Moreover, they can die. When we treat viral illness with viricidal drugs, our purpose is to kill viruses, much as we use bactericidal drugs to kill bacteria. And, perhaps most important of all, the powerful forces of evolution apply to viruses, just as they do to all other life forms. That is why it is so difficult to cure people infected with viruses. If a virus was nothing more than an inert collection of chemicals, there would never have been an AIDS pandemic. The human immune system would have mopped them up from the circulation without any difficulty.
It is clearly important that we take the trouble to understand viruses. We all know that this is important to medicine in combating viral illness in people. It is important also to veterinary medicine in combating diseases in animals, as it is to agriculture in combating diseases in plants. But there is another, even more profound, reason why we should take the trouble to understand viruses. My subsequent researches, and those of virologists such as Luis Villarreal and Marilyn Roossinck, have made it increasingly evident that viruses have played a key role in the evolution of life, from its very beginnings on Earth to the magnificent diversity we see today. Nowhere has the contribution of viruses been more significant than in the evolution of the human species. Perhaps most amazingly of all, this creative role in human evolution and disease has been played by viruses with a very close resemblance to HIV-1.
I realise that these will appear to be startling claims. When I first proposed such novel concepts, they provoked a heady mixture of bafflement and denial. The reaction was hardly surprising since, if I was right, it appeared to threaten the hegemony of the so-called “synthesis theory”, the trilogy of principles that has stood fast for more than seventy years as the theoretical foundation of modern Darwinism.
2
A Crisis in Darwinism
What [The Double Helix] conveys … is how uncertain it can be, when a man is in the black cave of unknowing, groping for the counters of the rock and the slope of the floor, listening for the echo of his steps, brushing away false clues as insistent as cobwebs to recognise that something important is taking shape.
HORACE FREELAND JUDSON1
A key proposition that has been almost universally misinterpreted among non-scientists as the core of Darwin’s theory is the concept known as the “survival of the fittest”. Nothing could have more alienated religious sensibility, with its potential for misapplication to society, for example its misuse in condoning laissez-faire politics in relation to poverty and hunger, and worst of all its extrapolation to racial and ethnic abuse. It is important, therefore, to clarify the fact that Darwin did not invoke the term. On the contrary, the concept of survival of the fittest was the brainchild of the social philosopher Herbert Spencer, who first proposed it in his book, Principles of Biology, published in 1864.2 Spencer had been developing his own thread of thought even before he read Darwin’s Origin of Species, which was published some five years before his own Principles of Biology, but the social philosopher was not educated in biology, and, although his concept was widely seen as synonymous with, or even a clearer exposition of, what Darwin was supposed to have meant by his term “natural selection”, Spencer misunderstood Darwin’s scientifically grounded theory, and he misapplied it as an endorsement of his sociological philosophy. The scientific historians, and philosophers, who have examined Spencer’s ideas have concluded that he saw evolution as a purposeful progression of the physical world, including all biological organisms, the human mind, and human culture and society. Unfortunately it was Spencer’s sociological concept of survival of the fittest, as opposed to Darwin’s scientific concept of natural selection, that led to the inaptly named Social Darwinism of the early nineteenth century, with all of its unfortunate ramifications.
There was never any true scientific foundation to Spencer’s ideas, but since they conveniently fitted with some of the prevailing prejudices of class, and the ethnic and racial bias of the late nineteenth century, extending into the first half of the twentieth century, they became deeply ingrained and influenced political and social belief. It is tragic that Spencer’s ideas still influence a lot of non-scientists today, so that one frequently hears the expression “survival of the fittest” raised in defence or excuse of some prejudicial action. So ingrained did Spencer’s ideas become that, during his lifetime, Darwin himself was put under a lot of pressure, by Spencer and others, to change his basic premise, but, although he briefly flirted with Spencer’s idea, he quickly recovered his senses and returned to his original concept.