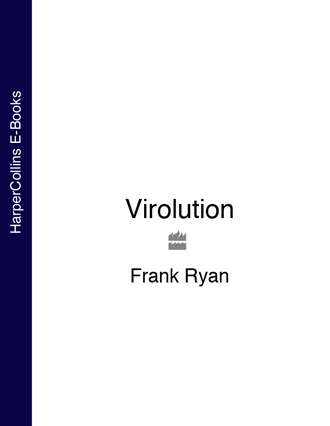
Полная версия
Virolution
Readers of Jan Sapp’s landmark history of symbiosis will discover how, in 1868, some nine years after Darwin had published The Origin of Species, a Swiss botanist, Simon Schwendener, made a curious discovery about the biological nature of lichens. We are familiar with lichens as the flat, pastel-shaded growths that decorate tombstones or the historic boulders of Stonehenge, but they are far more varied and ubiquitous than the cursory familiarity would suggest. They play an important role in the world’s ecology as pioneer organisms, thriving in inclement environments, such as sand-dunes or the windswept valleys of Antarctica, where they eke out a living on the exposed surfaces, breaking stone down into soil, or soaking up useful reservoirs of water from ambient dew or fog in forest ecologies. In this way, lichens create specialised ecosystems from which other life forms can benefit, for example the hardy growths that endure beneath the Arctic snow providing the main food source for the Sami’s reindeer. At the time of Schwendener’s discovery, lichens had only recently been slotted into place on the biological tree of life as a branch, in the jargon a “class”, of their own coming off the main trunk, or “kingdom”, of the plants, with naturalists devoting their time and energies to defining more than a thousand species that formed the twigs and leaves of that branch. Now, all of a sudden, such endeavour and certainty was thrown to the four winds when Schwendener demonstrated that lichens were not individual organisms at all but intimate associations of two radically different life forms, an alga and a fungus.
Since the time of Swedish naturalist, Carl Linnaeus, in the eighteenth century, biologists had assumed that all living organisms were discrete individuals, which existed as members of a species, which could be accurately assigned to its precise twig and leaf on the tree of life. We humans, for example, belong to the species sapiens, within the genus Homo, which is attached to the branchlet, or “order”, of primates, within the branch, or “class”, of mammals. But now it would appear that, rather than constituting any branch, or twig, or leaf, on the tree of life, lichens comprised an intimate intertwining of two of the main trunks – the kingdoms of the protists and fungi. For the orderly world of Victorian naturalists, the implications were devastating. Many lichenists refused to believe it and they roundly dismissed any such dualistic notions as an “abomination” that sowed confusion in place of order.
But despite the resistance, which endured in some quarters for almost half a century, study of the dual nature of lichens grew and spread, with some biologists, and botanists in particular, realising that lichens might not be the only example of an important association, or partnership, between very different living beings. This brought into sharp focus the concept of parasitism.
It was clear, from lichens, that the traditional idea of parasitism was inadequate to explain the real complexity of what studies were now revealing of the very close interdependency of the fungi and algae that made up the diverse group. Other examples of intimate interdependency of different life forms were duly recognised, from the coral reefs to forest oaks. In time the German botanist, Albert Bernhard Frank, would discover that virtually every plant was in partnership with a variety of fungi that fed into it, often physically invading the roots, so much so that the familiar root ball we shake out of its pot from the garden centre is largely fungus. The plant above ground supplies carbon compounds and energy to the fungus, while the fungus feeds water and minerals into the root. In the 17,000 or so species of orchids the relationship was so intimate that the fungus was found to supply the sprouting seed with carbon as well as water and electrolytes. The growing biological field demanded a formal name and definition and these were duly provided by another German botanist, Anton de Bary, who, in 1878, coined the term “symbiosis”, which he defined as “the living together of different organisms”.7 The definition was designed to embrace the many different associations already known to take place in nature, including parasitism, in which one of the partners gained at the expense of another, commensalism, where a partner gained without harming another, and mutualism, where more than one partner was seen to benefit from the relationship. The interacting partners became known as “symbionts” and the partnership, holistically, became known as the “holobiont”.
Over the years that followed, a dazzling variety of symbioses has been discovered in every ecological niche in nature, being particularly abundant in the flora and fauna of the oceans, including the very corals that manufacture the reefs, and the rainforests, with their fabulous diversity of life forms. It was assumed from the very beginning that such symbiotic relationships would have evolutionary implications for the participating partners, and in 1910 the term “symbiogenesis” was coined by the Russian biologist, Constantin Merezhkowskii, to define symbiosis acting as an evolutionary force.8
Today we recognise that symbiogenesis operates at several different levels. Most people are familiar with the cleaner station symbioses, where fierce predators, such as sharks and groupers, will patiently queue up at key sites on the ocean bottom and allow their skins, and even the interior of their mouths, to be cleaned of parasites and debris by smaller fish and shrimps. For obvious reasons this is known as a behavioural symbiosis. Metabolic symbioses involve the sharing of useful chemical products between the symbionts, as seen, for example, in the plant-fungal associations, or in the giant tube-worms, which inhabit the deep sea fissures, under the oceans. Here, along the volcanic summits, where tectonic plates are forming, the mouthless worms depend for their nutrition on symbiotic bacteria within their living tissues, and the bacteria, in turn, get their energy from the hydrogen sulphide that bubbles out of the “black smokers”. Many symbioses involve both behavioural and metabolic exchanges, for example the wide variety of pollination partnerships involving plants and insects, or hummingbirds, where the plant supplies the insects or birds with nectar, while the mobile partner carries pollen to other sedentary plants.
Symbiosis also works at a third, more powerful, level, where it is known as genetic symbiosis. This book started with a delightful enigma – the virally enabled transfer of genes necessary for photosynthesis across two kingdoms of life, as seen in the emerald-green sea slug, Elysia chlorotica. It would be surprising if biologists had not considered viruses as potential symbionts throughout the century or more that symbiology had grown and developed as a discipline. But readers will discover few references to viruses in Sapp’s book. In the decade after the Second World War, an American geneticist, Edgar Altenberg, had proposed a symbiotic “viroid” theory, based on the prevailing notions of the similarities of viruses to invisible “naked genes”, or plasmagenes, hidden in living cells. He conceived that viroids might have played a part in cellular evolution, and that cancer-causing viruses might arise de novo in every affected patient from viroids that had previously existed in the affected individual. Altenberg had conceived some startlingly original, even prophetic, insights – but he had been mistaken about the basic nature of viruses. Viruses are not naked genes. And his “viroid” concept was never embraced by the world of biology.
Ever the iconoclast, in the 1960s Rene Dubos also tried to persuade his virological colleagues to put aside their blinkered vision of viruses as nothing more than genetic parasites to consider that, in certain ecological conditions, they might sometimes enhance the host’s ability to survive. But back in the ’60s even the prescient Dubos had lacked the molecular technology necessary to prove his ideas to the world of science and so, once again, his colleagues had not been persuaded. From a wider reading of virological papers, I came across the occasional use of the term “symbiosis” in relation to viruses, sometimes with respect to the behaviour of whole viruses, whether infectious or incorporated into host genomes, and sometimes in relation to isolated genetic sequences derived from viruses. But none of the papers developed the term symbiosis in a way that would be accepted by the discipline of symbiology, which demanded a definition involving the interaction of living organisms, or life forms. The use of the term in relation to genetic sequences was clearly erroneous. And before we could even begin to make progress, from a definitional and developmental perspective, it would be essential to look very hard at the application of “organism” or “life form” to viruses.
Having taken Lederberg’s advice, I took pains over the years that followed to acquaint myself with how symbiosis was defined as a biological interaction, and in particular to how it worked as an evolutionary force. I read, and was enlightened by, the series of books and scientific papers of Lynn Margulis, distinguished Professor at Amherst, Massachusetts, who had played a central role in pioneering our understanding of symbiosis. I amassed a small library of other books, and papers, by symbiological colleagues throughout the century or so of the discipline’s history. I came to realise that many symbiologists misunderstood the essential nature of viruses, and this had given rise to erroneous assumptions, which in turn had delayed appreciation of their potential symbiotic role within the discipline. I thought I could see a way of accommodating the “organismal” or “life form” requirement. Even the most ardent of sceptics saw viruses as “coming alive” during their interaction with their hosts, and nobody denied that viruses were subject to the proven mechanics of Darwinian evolution. All biologists had to accept was that viruses should be defined in relation to their life cycles in their normal living ecology, and that such a definition allowed us to treat them as organisms or life forms from the evolutionary perspective – that small and seemingly reasonable step took me further towards a working definition that might be acceptable to both virology and symbiology. Through such research, and through a growing series of interviews with leading scientists within the two disciplines, I was in a stronger position to extrapolate a proven conceptual framework of symbiogenesis to viruses, and in particular to the potential contribution of viruses to symbiogenesis at genetic level. Meanwhile, it occurred to me that it might be useful to look at symbiosis from a Darwinian perspective.
Where Darwinian theory proposes an essentially linear pattern of evolution, with new species arising through branching divergence from ancestral stock, symbiosis involves a reticulate pattern of evolution through the partnership of different life forms, from species to whole kingdoms. On the face of it this would appear to suggest that symbiogenesis and Modern Darwinism have little in common. But this is not the case. In spite of the clear and important differences between the evolutionary mechanics that underlie the two evolutionary paradigms, symbiosis does not contradict evolutionary theory and it does not contradict Darwin’s concept of natural selection in particular. The crucial question we need to ask ourselves is not whether natural selection applies to the evolution of symbiotic relationships but rather how exactly it operates in circumstances where different life forms interact at a biologically meaningful level.
To put it bluntly – is there something different about the way in which natural selection works in symbiogenesis as opposed to mutation-plus-selection? Let us examine two familiar examples of symbiotic partnerships, and see if we can determine the answer.
Hummingbirds are native to the warmer parts of the Americas, where more than three hundred species depend on the nectar of flowers for their daily sustenance. The birds’ wings have been highly adapted by natural selection to allow them to hover, with pinpoint accuracy, over the flower, and their beaks have also become exceptionally long and shaped to fit the flower head, while their elongated tongues reach down into the well of nectar at the very bottom of the flower. Meanwhile, the flower has also been adapted to fit the bill of the hummingbird. One of the most striking examples of these birds is the violet sabrewing, which has a curved bill that fits the floral tube of its partner, the columnia flower, as accurately as a scimitar fits its streamlined scabbard. The precise match of bill and flower is important, since it deepens and strengthens the partnership, making it more likely that only the sabrewing will feed from the columnia, while the columnia’s stamens are positioned to dab pollen on exactly the right point of the bird’s forehead, so that it fertilises the next flower it visits. From this mutualistic symbiosis it is clear that selection is operating to a significant degree at the level of the partnership, stabilising and making permanent the living interaction.
If we turn our attentions to the behavioural symbioses of the cleaner stations, once again we see that these involve important changes in behaviour for both predators and cleaners: the predators put aside hunger and aggression, while the cleaner fish and tiny shrimps put aside fear and the instinct to flee. Such dramatic changes of behaviour in predator and potential prey would have to be hardwired into the genomes of the interacting partners and, just as we have seen with the hummingbirds and their floral partners, this involves each of the partners changing its behaviour in relation to the other. Once again we see selection operating at the level of the partnership in a mutualistic symbiosis. This also raises important questions about the real nature of viruses and their hosts. Could it be that selection might also be operating at the level of the virus-host interaction? If so, at what stage in the interaction did selection switch from operating at selfish, individual, even selfish gene, level, to recognise and begin to operate at this profoundly important level? This very question was addressed by the eminent evolutionary biologist, John Maynard Smith, late professor at the University of Sussex, and widely acclaimed as a pioneering Modern Darwinian.
In a chapter in the multi-authored book, Symbiosis as a Source of Evolutionary Innovation, which was edited by Lynn Margulis and René Fester, Maynard Smith developed a very interesting extrapolation of the Darwinian view of symbiosis. Believing that symbiosis played an important part in three of the five major transitions of life, he nevertheless insisted that there was no reason for symbiosis to challenge the neo-Darwinian view of evolution. But he also believed that, in order to accommodate the partnership aspects of symbiosis, there were circumstances in which natural selection must operate at a different level in symbiosis when compared with how it operates in the many Darwinian extrapolations.
It was even more helpful when, in exploring this further, he examined symbioses involving a microbial symbiont and a more complex host, and these he divided into various sub-categories. Where the symbiont could survive and reproduce independently of the host, this would suggest an evolution along conventional “selfish” Darwinian lines. But where the symbiont cannot survive without the host, and most particularly where the symbiont is dependent on the host for reproduction – a condition Maynard Smith termed “direct transmission” – the role of natural selection would inevitably change. Viruses can never survive, or reproduce, without their hosts. In this respect, viruses are said to be obligate parasites, so we should not be too surprised to discover that Maynard Smith included viruses in his discussion of symbionts that were only capable of reproduction through direct transmission.
In his words:
With direct transmission, the genes of the symbiont will leave descendants only to the extent that the host survives and reproduces. In general, therefore, mutations in the genes of the symbiont will be established by selection only if they increase the fitness of the host.9
When he writes that “mutations … will be established by selection”, he is referring to evolution taking place in the conventional Darwinian sense. Or to put it simply, the symbiont – the virus in virus-host interactions – will only be honed by mutation-plus-selection in a manner that increases the fitness of the host. In other words, the virus is now responding to the presence, and needs, of its symbiotic partner.
This interpretation of symbiosis, as seen from a Darwinian perspective, provides an important measure of common ground between the two disciplines. As we have seen in the examples of the hummingbirds and the cleaner stations, a symbiologist might adopt a slightly different perspective, regarding both host and parasite as symbionts, so that, rather than merely looking at the relationship from a single perspective, the symbiologist would examine how this might apply to the partnership. And all the evidence from what is now a weighty world of symbiology, with its study of a vast array of such partnerships, would imply that in microbe-host partnerships each of the partners responds to the presence of the other – or to put it from an evolutionary perspective, selection will be seen to operate, to a significant degree, at the level of the partnership. This perspective is seen to operate throughout all the levels of symbiosis, and, in the evolutionary sense, to symbiogenesis, whether at behavioural, metabolic or genetic level.
Within the genetic symbioses, there are examples of sudden and major change, where the genomes of radically different life forms unite to form a single novel, holobiontic, genome.10 In this very dramatic situation, which has the potential to give rise to very rapid evolutionary change, it is inevitable that selection will operate, to significant degree, at the level of the new holobiontic genome. It is perhaps not altogether surprising that some biologists see an irrevocable chasm in the evolutionary dynamics of this most powerful of genetic symbiosis and the gradualism that is assumed to be central to Darwinian evolution. But Maynard Smith does not agree. He goes on to emphasise that there is no contradiction between Darwin’s belief that complex adaptations arise by the natural selection of numerous intermediates, and the possibility that new evolutionary potentialities may arise suddenly if genetic material that has been programmed by selection in different ancestral lineages is brought together by symbiosis.
This is important not only in offering the potential of reconciling the dynamics of Darwinian and symbiotic evolution, but also in interpreting the role of symbiotic viruses in our human evolution.
4
The AIDS Dimension
In the summer of 1985, when a movie star was diagnosed with the disease … the AIDS epidemic became palpable and the threat loomed everywhere. Suddenly there were children with AIDs who wanted to go to school, laborers with AIDS who wanted to go to work … By the time America paid attention to the disease … the virus was already pandemic to the nation.
RANDY SHILTS1
Viruses have caused some of the great epidemics that project like tombstones through the fabric of human history. The quotation above is from the prologue of a book that chronicles the arrival of the AIDS pandemic in America, and the ensuing hue and cry of blame, prejudice, bewilderment, laudable dedication on the part of a few doctors and scientists and heartbreaking failings on the part of some politicians and key institutions, all conspiring to delay or limit the necessary response. It might seem counter-intuitive for me to suggest that even from such a grotesque tragedy we have discovered an important level of enlightenment. But this is speaking with hindsight and few, if any, understood the implications of this new, and utterly alien, organism when it first arrived on American shores in 1978.
In time we noted how, unlike smallpox, or pandemic influenza, the symptoms of AIDS conspired to mask its very arrival. It came among us stealthily, almost silently: the first entry of the virus into a new victim was all too often unrecognised, causing no more than a mild rash or fever – in half its victims causing no symptoms at all. After invasion, the virus continued to mask its presence, often hiding for years inside the blood cells known as lymphocytes, so that its victims remained none the wiser. Yet, even while hiding, it could be transmitted to other victims, through blood, through other bodily fluids, and particularly through sexual intercourse, heterosexual or homosexual. This parasite, and yes, I do suggest, aggressive symbiotic partner, is devoid of pity or prejudice, embracing all races, all ages from newborn to senility, and either gender. Meanwhile, throughout its period of silent invasion, the virus mutates at an extraordinary speed inside each infected person so that, some two or three years after first infection, the original strain of virus is no longer recognisable amongst the competing swarms of millions of different mutating progeny.
AIDS shocked society to its very core. Like a shudder come back to haunt us from the nightmares of smallpox and bubonic plague in the history books, it heralded a new face among Bunyan’s Men of Death. This plague was contracted in the main by sex amongst the young, and it was unlike any plague of old in the subtle and sinister way that it directly infected and killed the very immune cells that might best fight it off. Before the modern antiviral treatments, this destruction of the immune cells of the victim allowed all manner of secondary horrors to invade, so people drowned in amoebae, normally inconsequential germs from tap water that invaded their bowels and spread into their internal organs; or strange viruses, such as the cytomegalovirus, hopped a ride in a vicious parody of their normal patterns of infection. The skins of sufferers were showered with purple-bluish cancers. Bizarre forms of leukaemias coursed their bloodstreams. Every surface of their bodies, both skin and internal, was tormented and disfigured by an anguish of afflictions. It was a vision of hell the equal of anything dreamed up in Dante’s Inferno. To make matters even worse, the plague could be passed from a mother to her children, so the horror was handed down, like some nightmarish legacy, to the next generation. This was a particular problem in developing countries, when either one or both parents died, leaving the sick children to be cared for by devastated grandparents, or handed over to ill-equipped orphanages, or abandoned altogether.
There are two causes of the AIDS pandemic, related retroviruses known as the human immunodeficiency viruses, HIV-1 and HIV-2. Perhaps at this stage I should explain that retroviruses are a large and complex family of viruses, all of which have in common the fact that their genes are made not of DNA, as are all other life forms other than viruses, but of its sister molecule, RNA. The retroviruses infect virtually every animal species, from the simplest of marine invertebrates – such as sea slugs – to primates, including humans. As part of their normal infectious life cycle, they need to inject their viral genes into the nuclei of their hosts, so it is vitally important to understand just how they do so. Retroviruses usually spread through mating, or from mother to baby in a variety of ways. The HIV-1 virus is transferred in the pre-ejaculate male fluid and in the vaginal fluids that help to lubricate normal sexual intercourse, in semen, in blood – for example, through contaminated needles, contaminated blood transfusions, through rough intercourse, or during the delivery of a baby – and also in the mother’s milk during breast-feeding. The virus finds its way into the blood, from where it discovers its primary host cells, mainly cells involved in the immune system, known as helper T-lymphocytes – the technical term is CD4+ T cells – but it will also multiply in other cells, such as the white blood cells known as macrophages, and in various other tissues and organs of the body, especially the testes. After entering the T-lymphocyte, the virus uses its own enzyme, reverse transcriptase, to convert its viral RNA genes to the equivalent DNA genes, and then it inserts its entire genome, in this DNA form, into the chromosomes of the lymphocyte. This process, which is typical of all retroviruses, was discovered by the Nobel Laureate, Howard Temin, who called this DNA form of the virus the “provirus”, which also includes the dynamo regulatory regions known as LTRs. It stays in the chromosomes for the lifetime of the infected cell, and is reproduced within the chromosomes every time the infected cell divides to form daughter cell offspring.