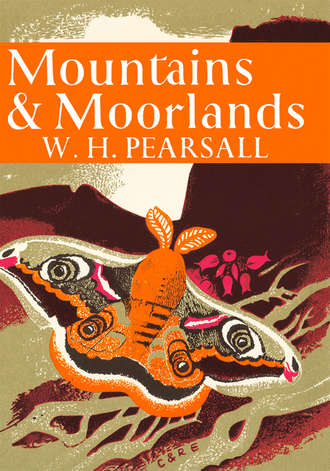
Полная версия
Mountains and Moorlands
Much British mountain scenery is that characteristic of a glaciated and ice-eroded country. That there is a marked contrast with other regions will be at once apparent if one compares a typical British upland scene with one, for example, from the Grand Canyon of Colorado.
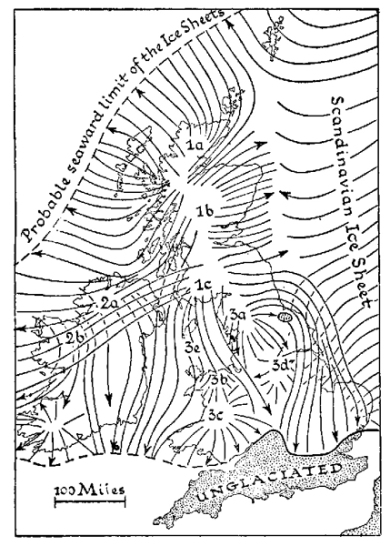
FIG. 7.—Ice movements in the British Isles. GLACIATION
The most striking feature in the recent geological history of the British Isles was the series of great Quaternary Glaciations, which terminated only some 10,000 years ago. For biologists this is a convenient starting-point for recent biological history, but it was scenically of equal or greater importance. In order to obtain a picture of what Britain was like during the Glacial period, we should have to try to imagine it buried beneath a great ice-sheet many hundreds of feet thick, and covering, at its maximum extent, almost the whole of these islands north of the River Thames. The centres of ice formation were the areas with greatest precipitation (then snowfall, now rain), particularly the greater area of the Highlands of Scotland, centring on Rannoch Moor, to a less extent the Southern Uplands from Merrick outwards and the smaller Lake District, and also, but still less, Snowdonia. From these and other smaller centres the ice flowed outwards, though very slowly. A huge existing ice-sheet, that in Antarctica, to-day is still moving at the rate of a yard and a half a day when it reaches the sea as the Ross Barrier, hundreds of miles from its source.
We can trace the main directions in which our British ice-sheets moved, because they carried with them all the soil and rock detritus that had accumulated on the surface of the land in the preceding ages. Any unusual types of rock are readily recognised and, because they have characteristic fossils or special mineral constituents, limestone and igneous or volcanic rocks are especially useful for this purpose. Rocks thus found far from their place of origin are termed erratics, and the photograph in Pl. VI, shows a well-known example, one of the Norber boulders in West Yorkshire, slate rocks carried by ice from an adjacent valley and left on top of the Carboniferous Limestone which normally overlies the slates (see Fig. 2). In England, erratics of the Shap granite, coming from a small area in the eastern Lake District, have been particularly valuable in tracing the movements of Lake District ice. A magnificent boulder of this rock some ten feet in cube, standing in the main quadrangle of the University of Manchester, illustrates the fact that ice from the Lake District left debris as far south as Cheshire. Farther west there was an ice-flow carrying Galloway granite to Flint and Shropshire. Similar evidence shows that some Lake District ice went east over Stainmoor, leaving boulders of Shap granite as far away as the Yorkshire coast. The accompanying map (Fig. 7), constructed mainly from evidence of this type, shows the main lines of ice movement in Britain during this period. It will be noticed from this map that the ice movements did not always follow the obvious lines of outward radiation. In Lancashire and Wales, for example, the ice was deflected southwards and eastwards by Scottish and Irish sea-borne ice. In Scotland particularly, and to some extent in Yorkshire and Northumbria, the outward-moving ice was dammed up and deflected by Scandinavian ice coming across the North Sea. Moreover, in the partial northerly deflection of the northern ice there is evidence that it overrode mountains 3,000 ft. high. On the west coast of the Highlands, the ice-marks not only reach this altitude, but, allowing for the depth of adjacent lochs, it can be estimated that the ice-field must at times have been some 4,000 ft. thick. Similarly in the Lake District, where the area of high precipitation is much smaller, the ice-fields were some 2,000 ft. thick. Indeed, on Scafell and Helvellyn the marks of glaciation may be seen up to a height of 2,500 ft. It is not easy to imagine the scale of this ice-covering. The nearest thing to it at present may be the Greenland ice-cap; that in Antarctica is apparently larger.
Even at its maximum extent, it did not wholly cover the country (see Fig. 7). There could not have been much ice south of the Thames, and Dartmoor seems to have been quite unglaciated. Moreover, the highest mountains, and, indeed, many of the lower outlying ones, projected through the ice as nunataks. They can often be recognised by their greater altitude and bolder shape, which contrasts markedly with that of the lower, rounded and glaciated hills. In the later stages of the Ice Age, at least, considerable areas of the Southern Pennines may have been generally ice-free, though no doubt supplied with local snowfields. The main ice-flow at this time seems to have been deflected by the Howgill and Bowland Fells, or westward down into the Cheshire Plain. The existence of ice-free areas makes the comparison with Greenland more valuable and it allows us to assume that there were probably at least some plants and animals there.
In their movements, the ice-sheets not only scoured away existing soils and rock debris, but they also scraped away rock. Thus in glaciated regions, every projecting rock tends to be smoothed and scratched on the exposed side, even if it retains rough surfaces on the lee side. Such rocks are termed “roches moutonnées,” and often they allow us to infer the direction of local ice movements even better than do erratics. Although it did not invariably do so, the moving ice tended to follow existing valley lines and hence these were scoured out and deepened, particularly towards the valley heads where the ice was normally deeper. Often rock basins were formed which now contain lakes (see Pl. 8). The form of these glaciated valleys (and of the lakes) is very characteristic: they tend to be “canal-sided” in plan and U-shaped in section. The effect of these great ice-sheets is not only to deepen and broaden the main valleys but also in doing so to remove the lower and gentler slopes on each side. Thus spurs are cut off and lateral valleys are cut short, while the lateral streams they contain now tend to enter the main valley by sudden rapids or waterfalls. “Hanging valleys” of this type and “truncated spurs” are a characteristic feature of British mountain scenery. The photograph of Loch Avon in Pl. 8, shows a fine example of a hanging valley, while truncated spurs can be seen in Pl. 10.
Additional results of this form of erosion are, first, that vast quantities of detritus are removed and scattered over the adjacent country; and, secondly, that the existing forms of low relief are “sharpened” as it were and made more mountainous in aspect (see Pl. 23). The gentle plateau-like profiles of some of our mountain areas, as seen from a distance, give no hint as to the steepness and wildness of the ridges and valleys they prove to contain. At the same time, the removal of pre-existing soils and gentle slopes has generally left the valleys in what is essentially a “montane” condition of bare rock or rock detritus in marked contrast to the deep and long-established soils that must have prevailed in pre-glacial conditions.
As to the materials left behind by the ice, the most obvious are usually coarse rock-waste in the valleys, often material which had fallen from adjacent hills on to the ice during the last stages of its retreat. The effects of these last remaining valley glaciers were comparable indeed to those observed in existing high alpine regions, but they were insignificant as compared with those of the great inland ice-sheets. The detritus accumulated by these sheets in their ground moraines and left behind when they finally melted, has largely determined the appearance of the existing British lowlands and indeed of the lower valleys, obliterating all pre-existing soils or underlying rock, and often smothering the pre-glacial features under layers of “drift” 30 or 40 ft. thick (see Pl. V). Although it may contain rock-waste of almost every type, the drift usually consists of some form of boulder clay in which ice-worn and scratched boulders are mixed with what is really rock-flour. The prominent clay fraction of this drift, whatever its general texture, usually suffices to make it “set” readily, and accordingly it often tends to be impervious to water. Not only has this type of material been scattered to great depths all over the lowlands adjacent to our mountain areas, but, in thinner sheets, it will also often be found to be plastered over almost any area of low relief in the mountains themselves. Consequently most gentle slopes in the upland districts, if not left scoured clean by ice action, are covered with drift which is often of a clayey nature.
These drifts and morainic deposits usually contain material carried from a distance and, like the erratics, unrelated in characters to the locally underlying rock. Pl. 9, for example, shows some glacial ground moraines of a non-calcareous nature bearing moorland vegetation, although the underlying rock is limestone, on which such vegetation does not normally occur. A similar effect is clearly shown in Pl. 17, where drift overlying limestone has blocked up the drainage so thoroughly that peat, bearing moorland vegetation, has developed over the limestone. Of course the reverse can also take place. In many places, calcareous clays have been distributed over non-calcareous strata, and thus as a general rule considerable caution is necessary in glaciated regions in attempting to relate soil or vegetation types to underlying rock.
Of course, at the close of the glacial period, there was a great deal of resorting of the rubbish left behind by the melting ice. The vast quantities of moving water which must have resulted during the melting process obviously redistributed the morainic materials on an enormous scale. Consequently to-day we often see streams running down valleys that now seem far too large for them or issuing across deltas which, quite obviously, they could never have produced in their present condition. A particularly striking condition at that time must have been the numerous large lakes held up among the ice-sheets, often in what are now quite unexpected places. At least one of the high limestone hills in Yorkshire, Moughton Fell, has lake silts on its summit, and such sediments or delta cones deposited in water are not uncommon on the flanks of the wider valleys. A classical British example, Lake Pickering, lying to the south of the Cleveland Hills in North Yorkshire, is associated with the name of the late Professor P. F. Kendall, who showed how the existence of the ice-dammed lake could be recognised. While we are not concerned here with the detailed history of these bodies of water, it should be recognised that they left behind various sediments, including impervious ones, that helped to create local stagnation of the drainage system; and, as we shall see, so gave a definite character to the sites they had occupied.
The great glaciation merits more attention than we can really give it, and this not only because it moulded our scenery. It is far more important because it represents a characteristic phase in the history of our mountains and moorlands as we now know them, as well as the agency which has perhaps more than anything else determined their present biological character. Whatever may ultimately prove to be the underlying cause of such an ice-age, it cannot effectively develop, as Sir George Simpson has emphasised, without the heavy precipitatation (then as snow, now as rain) that characterises British mountains. From this point of view, the Ice Age seems to be as characteristic of British highlands as is the present climate. From the historical point of view, the Ice Age is important because it means that the starting-point from which our present fauna and flora is derived must have been largely an arctic-alpine group of organisms. From still a third point of view—of particular interest to the botanist—the glacial epoch left behind it an upland country largely sterilised by the removal of existing soils and fertile deposits. Some areas, such as large parts of the Hebrides and of Sutherland, were in fact left sterile, and even to-day remain as an almost bare and undulating rock surface occupied only by small tarns and moorland of the bleakest type. This is a common condition among the mountains. But speaking generally, even among the mountains and particularly among the foothills round each mountain group, areas of gentle slope were usually plastered over with clayey drifts or sediments. The result was a great deterioration of the natural drainage in many areas. Even the limestone hills, as we have seen above, Plates 9 and 17, though naturally extremely porous, often suffered in this way, and in many cases cannot be superficially distinguished from the non-calcareous rocks around them in this respect. Elsewhere, and particularly in the valleys, the erosion of the lower slopes by ice and by glacial streams left behind a sharpened relief of a much more montane character and, incidentally, often paved the way for a new cycle of erosion.
RECENT EROSION
The forms of the mountains we see to-day are clearly the results of three agencies — of the original rock structure as modified by large-scale earth movements, of the long continued erosion which, acting on the original structure, has fixed the positions and outlines of the main valleys and summits, and, lastly, of the sharpening of land forms and the removal of pre-glacial screes and soils by ice action. As a habitat for living organisms, the surface of a mountain is as important as its skeleton, and this is affected not only by the legacy of slope and structure already described, but still more by the recent or post-glacial effects of erosion. Speaking generally, the upland surfaces are either physically stable or unstable, and it is the large proportion of unstable surfaces which is particularly characteristic of upland areas. Nevertheless, even the stable surfaces, those more comparable in slope and form with lowland areas, show peculiarities, for they are often rock surfaces, either scraped clean during glaciation (as in the Northern Scottish examples just mentioned) or sometimes, like limestone pavements, composed of rock which yields little or no soil on weathering. Even the soil-covered stable surfaces are often areas covered by poor glacial drifts or with impervious rock strata beneath, and now mostly peat-covered.
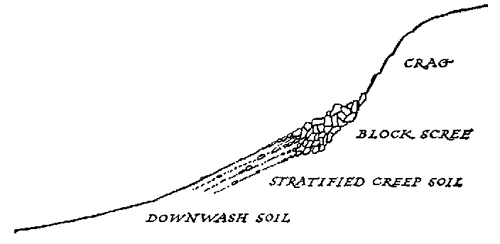
FIG. 8.—Arrangement of main zones below a rock outcrop.
The unstable surfaces naturally tend to lie along the main lines of erosion, and they include both the places over-steepened by ice action as well as those showing the immediate effects of stream erosion. The most widespread type of unstable surface is the steep scree-slope with its capping of crag. This generally shows a gradation of form and composition such as is illustrated in Fig. 8. The upper part is usually the steepest, and consists of coarser detritus, while the lower part shows finer detritus and gentler slope. As on any steep slope, rainwash leads to the accumulation of the finest materials on the lower parts.
The downward movement of material continues long after an angle of primary stability (usually between 30° and 40°) is reached; and there are numerous interesting manifestations of this movement. The larger stones, in particular, are usually persistent “creepers,” expanding more on the lower side when the temperature rises and contracting more on the upper margin when cooling takes place. They often continue to move downwards long after the rest of the surface has been stabilised by vegetation. When such stones are elongated in shape they generally tend to progress with their long axes more or less parallel to the slope, as may be seen in Pl. 25. Although the larger boulders move most persistently on partly stabilised screes, they usually move more slowly than the finer material on loose scree slopes, where the finer materials often accumulate around the upper side of the boulders, giving a step-like arrangement. Obstacles such as tufts of grass lead to a similar effect, so that some form of terracing is particularly characteristic of steep mountain slopes, even after they have been partly stabilised by vegetation, and one has only to look down on a steep grassy slope under suitable lighting conditions to see what are apparently innumerable more or less parallel “sheep-track” terraces, due mainly to the agencies of soil-creep and rain-wash, though nowadays much accentuated by the movements of grazing animals.
While the characteristic features of crag and scree may occur at almost any level, there are other types of instability which are particularly characteristic of the higher altitudes above 2,000 ft., and generally most clearly shown on the high summits. The high mountains are generally but little affected by the action of running water, and their erosion is due far more to the effects of frost and snow, sometimes collectively distinguished as nivation.
The surface of the higher and steeper summits is commonly covered with rock detritus, sometimes to a depth of several feet (see Pl. III). This material, often called mountain-top detritus, is formed by the disintegration of the native rock by the action of frost. The size of the individual fragments, as in the case of screes, depends largely upon the hardness and the physical character of the underlying rocks.
The frost detritus or mountain-top detritus is the most characteristic of summit surfaces. Its appearance is well illustrated in a number of the plates included here: Pl. 11a Pl. 12 Pl. 25 and its loose surface indicates the constant struggle between the stabilising effect of vegetation and the instability due to wind exposure and the action of frost, snow and gravity. In the plates given here, the striking instability of very slight slopes at high levels is clearly shown. In the examples pictured in Pl. 11a and Pl. 25 the slopes have an inclination of only about 10° to 15°, although the surfaces show little tendency to be fixed by vegetation. A slope of 30° at lower altitudes would quickly become completely covered by vegetation and hence more thoroughly stabilised.
The instability of the surfaces at high altitudes is not confined to those that are predominantly or wholly stony. It is equally evident on many of the more rounded mountains (“moels”) and on those on which the friable nature of the underlying rock has permitted some soil formation. Here solifluction effects may become extremely marked. When soil highly charged with water first freezes and then melts, the expansion accompanying freezing makes the soil very unstable when it thaws, so that downward movement on even the gentlest of slopes becomes possible, the semi-fluid surface slipping easily over the frozen sub-soil. On steeper slopes, large volumes of muddy detritus may be stripped off the flank of a mountain through this agency, and at high levels soil-covered slopes, however slight, almost invariably show signs of movement produced in this way (see Pl. XIb). The most frequent signs are different forms of terracing, and these occur on quite gentle slopes and where vegetation is present. The swollen soil behaves almost as a series of fluid drops, each partly restrained by the turf, which prevents complete movement, bounding the whole on the lower side in the form of a step, the earth being exposed on the upper flatter part.
Of the reality and importance of these influences, no one who has frequented mountain summits in spring can have any doubt. The mountain soils at that time are “puffed up,” as it were, so that the foot sinks deeply into them. The frequent freezing and thawing has the effect of mixing the soil surface, and, in particular, it causes frost-heaving by which the stones present are extruded, so that the surface is commonly more stony than the material beneath.
The processes seen at work on the higher mountain summits bear a considerable resemblance to those observed in arctic regions. On flat or nearly flat surfaces in the Arctic, solifluction effects are associated with the production of curious “stone polygons” in which a central area of mud, often associated with smaller rock detritus, is surrounded by a polygonal boundary of larger stones. Possibly because of the prevalent slopes, polygons of this sort are not very common on British mountains, although they have been recorded by Professor J. W. Gregory from Merrick in the Southern Uplands and by Dr. J. B. Simpson from Ben Iadain in Morven. An interesting area may be seen at about 3,100 ft. on the broad saddle connecting Foel Grach with Carnedd Llewellyn. This shows that the polygons are found only on a flat surface, giving way to “stone stripes” as soon as the
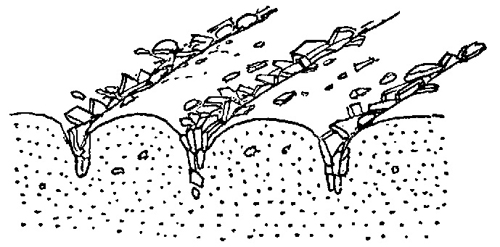
FIG. 9.—Distribution of materials below “stone stripes.” (Diagrammatic.)
surface acquires an appreciable slope. Stone stripes, or the somewhat similar “striped screes” which appear in coarser and more sloping material, were first described in this country by Professor S. E. Hollingworth from examples in the Lake District, where, once one learns to look for them, they are not uncommon. The larger stones collect in rows parallel to the slope as is shown in Fig. 9. The stone stripes, like polygons, overlie soil, and presumably the stones have been extruded from the soil by the movements due to freezing and thawing. Apparently both polygons and stripes occur where frozen layers of soil persist below the thawed surface. The British mountain polygons and stone stripes are often quite small. Those shown in Pl. XI, were only about a foot apart, though where the movements are on a larger scale they may be three or four times this size. There are especially striking ones on the eastern face of Yr Elen in Snowdonia which can easily be seen from a distance of over a mile.
Many of these solifluction areas illustrate the general feature that the unstable areas on high mountains are often as characteristic of gentle slopes as of steep slopes. Thus the average angle at which an equivalent degree of stability is reached seems to be much less at 2,500 ft. and upwards than at, say, 1,000 ft. No doubt the slower growth of vegetation at higher altitudes also contributes to this condition. Nevertheless, if it is invariably the case, the difference must have a considerable influence on the shape of a mountain. Wherever the rock structure allows comparable rates of weathering, we might perhaps expect to get a shape of the type illustrated in Fig. 4, rather than the simple cone. The preliminary steepening of the lower slopes must, of course, be due to more remote causes.
There are probably other processes by which rock and soil movement may be brought about at high levels, though they do not seem to have been much studied in this country. Some are undoubtedly associated with places where snow lies long. Where such a slope persists below a region of surface instability, rock-waste may move rapidly downward across the snow surface, collecting in a band at its base. Further, long-persistent snow-banks almost always terminate below in erosion channels, which, at the higher levels, may give permanent drainage channels cutting back towards the mountain crest. The interest of these features is not only biological (see here), but it lies also in their possible bearing on the origin of the high-level corries (or cwms or cirques) so often found in the larger British mountains. In the extreme form these are rock basins and undoubtedly relics of the small high-level glaciers and nevé which must have lingered on for long after the main ice-sheets had passed away. Corries seem to be most frequent on the east of a main summit or ridge, and it may be that in the first place their position was the result of a semi-permanent snow-bank which started an erosion system. In the later stages it has been supposed that the upper nevé exerts a plucking action on the frost-shattered mountain face, through the periodic filling and downward contraction of the bergschrund, if one may use this term in such a case. In this way continuous over-steepening of the head of the erosion system may have resulted in the formation of the crags encircling the corrie. It seems probable that corrie-formation was most vigorous during and just after the Ice Age, but as it usually lies above the other main erosion effects of the ice-sheets it may be appropriate to regard it as an extreme effect of persistent snow-lie.