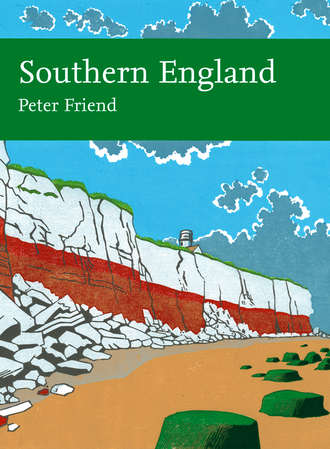
Полная версия
Southern England
Continuing research into the processes operating within the Earth shows that movements of the Earth’s crust are taking place continuously, even though the rates involved are generally too slow to be noticeable. The discovery that the Earth’s surface consists of a large number of tectonic plates in continuous relative movement was one of the major breakthroughs in the earth sciences, and has fundamentally changed our understanding of the planet. More on this topic will be considered in Chapter 3, but at this point it is important to realise just how slow the rates of movement are: at most a few centimetres per year on average (often compared to the rate at which fingernails grow). Occasionally, movements of centimetres or metres occur within seconds along faults during earthquakes, but the average rate of movement is still rather slow. Most of us living in stable areas are totally unconscious of any movement at all because we are, ourselves, moving slowly with the landscape that we live on. Slow though the movements may be in a particular landscape, so also are the rates of surface modification, and the balance between the two is a delicate one. In much of Southern England modification by surface processes is dominant, but this has not always been the case.
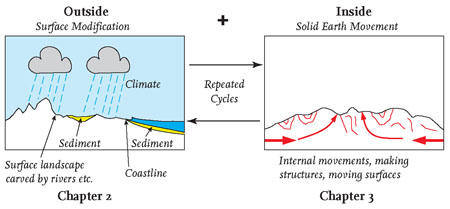
FIG 5. Landscapes are changed by surface modifications (Chapter 2) and solid earth movements (Chapter 3).
Our next chapter deals with the timescales represented in the landscapes of Southern England and the processes that have been modifying them. Chapter 3 deals with the movements from below – from within the Earth’s crust – that are ultimately creating major landscape patterns.
CHAPTER 2 Time, Process Southern England’s Landscapes
BEDROCK AND SURFACE BLANKET
WALK AROUND THE COUNTRY IN SOUTHERN ENGLAND and the ground beneath your feet is very rarely solid rock. You are walking over soil made of weathered mineral grains and organic debris, along with other relatively soft and granular materials that make up the surface blanket. Beneath the surface blanket lies solid rock, the bedrock of the landscape.
Bedrock forms the bones of the land. From the colour of the soil, to the elevation of the hills, to the types of vegetation present, the landscape is profoundly influenced by the bedrock underlying it. For example, in Southern England the Lower Greensand (a distinctive layer of bedrock of Early Cretaceous age, see page 26) produces soil water with acidic chemical properties. The Lower Greensand was originally deposited as sand over a period of a few million years, more than 100 million years ago. This layer represents a different environment of deposition from the older sediments on which it lies, and was followed by another change of environment which produced the deposits that lie on top of it. Both the preceding and the following bedrock deposits have alkaline chemical properties. In certain regions the bedrock layers have now been brought to the surface of the landscape by erosion and movements within the Earth. The Greensand is harder than the layers above and below it (largely mudstones) and so is generally more resistant to weathering. In some areas the Lower Greensand lies just below the surface blanket and has resisted the general landscape erosion to form a distinct Greensand ridge running across the countryside, characterised by special vegetation adapted to the acidity of the soils.
It is only in cliffs or at man-made excavations such as quarries that we can see bedrock at the surface in most low-lying areas. By using those areas where the bedrock does outcrop at the surface, and the results of drillings (e.g. for wells), we can discover the types and arrangements of rock below any landscape.
THREE DIFFERENT TIMESCALES
More recent past events tend to be better known and of greater interest than distant past events. Figure 6 is plotted on a logarithmic timescale, so that the most recent times are given more space and greater ages are given less and less space.

FIG 6. Three different timescales, plotted to give more space to more recent events.
For the purposes of this book, we can distinguish three overlapping timescales to help us to understand the landscapes of Southern England:
The bedrock timescale (extending from 542 million years ago to about 2 million years ago)
The Ice Age timescale (covering roughly the last 1 million years)
The last 30,000 years timescale
We shall now review each of these, commenting on the sorts of episodes in each that are important in our exploration of Southern England.
THE BEDROCK TIMESCALE
Figure 7 is a simplified version of a generally accepted geological timescale relevant to the landscapes of Southern England. The names of the divisions are universally accepted in the geological world and, unlike the previous diagram, the passage of time is represented on a uniform (linear) timescale. The divisions have been selected, and sometimes grouped, to help in our analysis of the situation in Southern England, and these have been colour-coded for use in the rest of the book.
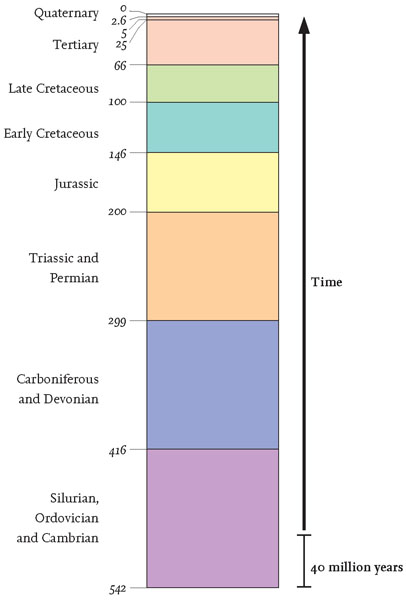
FIG 7. Bedrock timescale for Southern England.
The rocks at, or just below, the surface of Southern England range in age over hundreds of millions of years, and most of them were formed long before the present scenery began to appear. At the time of their origin, these rocks were deposited in a variety of different environments, mostly when mud or sand materials were transported into and/or around the seas that existed where England is now. Most of the bedrock of Southern England was formed in this way and is said to be of sedimentary origin. The depositional conditions varied from time to time: the climate varied, the geographical pattern of rising and sinking land movements changed, and the supply of mud and sand brought downstream by rivers changed also. Despite these fluctuations, it is possible to generalise the way that sediment has accumulated over an area the size of Southern England, and to offer a succession of layers of different composition, age and average thickness that can provide a general guide. This is shown in Figure 8.
For each of the Regions (and some of the Areas) discussed in Chapters 4 to 8, a rock column, generalised for that particular area, will show the main bedrock layers. Each column will be coloured using the standard colour codes of this book to represent the ages of the layers.
As an example, we will consider another particularly distinctive layer of bedrock, the Chalk, which ranges between 200 and 400 m in thickness. Chalk is visible quite widely at or just below the surface over perhaps a quarter of the area of Southern England (Fig. 9). Chalk is an easily recognised rock because it is made of very small fragments of lime (calcium carbonate) and is usually brilliant white. It formed from fine-grained limey mud deposited on the sea bed, but through many millions of years of burial below other sediments it has been compressed and altered into the hard rock we recognise today. The Chalk is a result of a unique combination of environmental conditions and the presence of particular algal organisms in the history of evolution. It is only found in northwest Europe, and was only formed in Late Cretaceous times.
The presence of Chalk near the surface in Southern England is almost always linked to the presence of hills and slopes in the scenery, clearly showing that Chalk is a tough material that resists landscape erosion more than most of the other rock types available. The Chalk is also noteworthy because it represents the most recent time when most of Southern England was covered uniformly with soft sediment and a shallow sea: in Late Cretaceous times, except for possible islands in the southwest, there was no emergent land across Southern England.
Like all sedimentary bedrock layers, the Chalk initially formed as flat layers or sheets of sediment, extending widely across the floor of the sea. As will be discussed in the next chapter, these sheets of sediment are generally characteristic of stretching movement episodes in the Earth’s surface. Such movements produce areas of collapsed and low-lying land that can accommodate large volumes of sediment, if it is available.
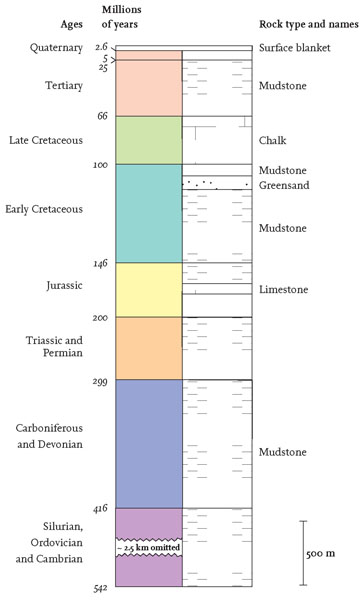
FIG 8. Generalised succession of the bedrock of Southern England, showing a typical thickness for each layer.
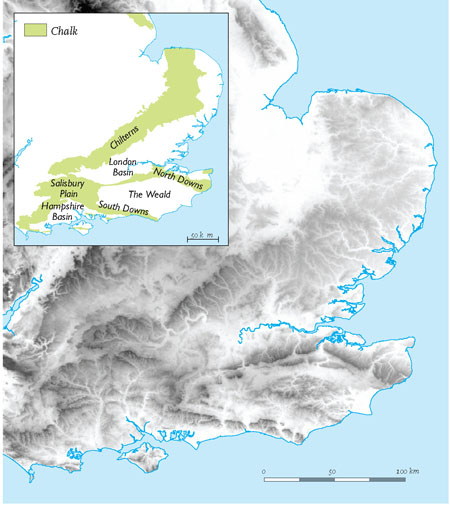
FIG 9. The Chalk and its topography. The darker areas represent the chalk uplands.
However, we do not see the Chalk at or near the surface everywhere across Southern England; instead the Chalk forms narrow bands across the land. This is due to later movements affecting the bedrock layers by folding and tilting them, so that some parts were raised (to be later removed by erosion) and other parts were lowered (Fig. 10, A and B). In the millions of years since the sediment layers were laid down, they have been buried, compacted, deformed by various processes, and finally uplifted to form part of the landscape that we know today (deformation processes are treated more fully in Chapter 3). The Chalk layer has been moved and folded as a result of mild compression or convergence, to form a downfold or syncline between the Chilterns and the North Downs, and an upfold or anticline between the North and South Downs (Fig. 10, C). Later, the central part of the anticline was eroded away to produce the bedrock pattern that we recognise today (Fig. 10, D). The vein-like river valleys visible on the elevated Chalk hills of Figure 9 are evidence of this continuing erosion.
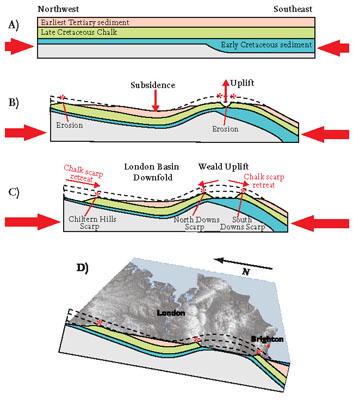
FIG 10. Deposition and folding of the Chalk.
LANDSCAPE MODIFICATION BY RIVERS
Weathering of landscape surfaces and the production of soils by the action of rainwater, air and organisms are important factors in shaping landscapes. These processes affect the bedrock when it is very close to the surface, and most of them weaken the material that they work on. This is particularly so when tough silicate rock minerals are altered to soft clay minerals, which are then easily eroded. Freezing and thawing also works to weaken the bedrock as water in cracks freezes and expands, breaking the rocks into fragments.
Whilst weathering is a widespread and general process, most of the other important landscape processes involve the formation of discrete features that we shall call landforms. Rivers result in the formation of a number of important landforms that are described below.
The most important landforms resulting from river processes are the channels of rivers and streams (Fig. 11). When rain falls onto a land surface some of it soaks into the land (forming groundwater), whilst the remainder runs along the surface, collecting in topographical lows and producing stream and river channels. Today, many of Southern England’s river channels tend to be relatively narrow and shallow – only metres or tens of metres in width and less in depth – so they occupy an extremely small percentage of the area that they drain. However, they are still the dominant agents of landscape change, causing downwards and/or sideways erosion as well as acting as conduits to transport the eroded material out of their catchments.
Most river channels develop a sinuous course, becoming curved (or meandering) to varying degrees, or developing a number of channels separated by islands of sediment (becoming braided). The positions of the curves or islands change with time as sediment is shifted downstream, and the position of a river channel will change with time correspondingly.
Because of their ability to erode material and remove the resulting debris, river channels create valleys. The sides of a river valley are referred to as slopes. When a channel cuts downwards the valley sides generally become steeper and slope material (generated by ongoing weathering processes) moves down-slope towards the channel. The material is transported either as small individual fragments or as larger mass flows. Where down-slope movements involve the collapse of large areas of material, the terms landslip or slump are often used. Slope material is then deposited in the channel and removed downstream by the river.
The simplest valleys result from down-cutting by a river or stream to yield a V-shaped profile in cross-section. The gradient of the valley sides depends on the strength of the material that the slopes are composed of in the face of erosion. Stronger materials are more difficult to erode and remove, and so can form steeper slopes than weaker materials. In some areas, the river channel is unable to form valley slopes as the material is too weak to form a noticeable gradient. In the Areas we will be investigating, it is clear that some of the slopes are largely the result of a particularly strong layer in the bedrock resisting erosion as the landscape has developed.
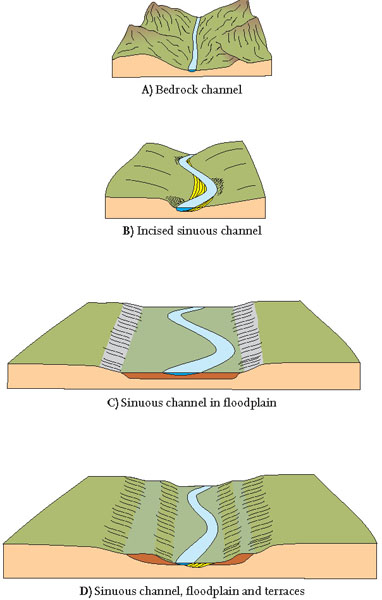
FIG 11. Landforms of rivers.
As the valley develops, its profile can become more complex. In some cases, slopes appear to have retreated across a landscape some distance from the position in which they were initially created by river down-cutting. A river with a wide valley floor is one of the most obvious examples of this, in which movements of the channel across the floor have caused the slopes to retreat as the valley floor has become wider. In some cases, slopes appear to have retreated over many kilometres from the original valley as numerous collapses of the slope took place.
Overall, therefore, the valley profile and the channel course reflect variations in the strength of the material being eroded, and in the strength and flood pattern of the river. Climate changes are likely to have a major effect on the strength of the river by altering the volume of water flowing through the channels. Additionally, the lowering or raising of the channel by Earth movement effects (see Chapter 3) can affect the evolution of the landscape by river processes. For example, both climate change and the vertical movement of the river channel can initiate the formation of river terraces. Different examples of all these river geometries will be discussed in greater detail in the Area descriptions in Chapters 4–8.
Over millions of years, river down-cutting, slope erosion and material transport tend to smooth and lower landscapes until they approximate plains, unless they are raised up again (rejuvenated) by large-scale Earth movements (Chapter 3) or are attacked by a new episode of channel erosion, perhaps due to climate or sea-level change. Southern England generally has a smoothed and lowered landscape, representing hundreds of thousands of years of this river and slope activity.
The branching, map-view patterns of river channels and valleys are an obvious feature of all landscapes. An approach to understanding how this forms is illustrated by a computer-based experiment (Fig. 12) in which a flat surface (plateau or plain) is uplifted along one of its edges, so that it has a uniform slope towards the edge that forms the bottom of the rectangle shown. Rain is then applied uniformly across the surface, causing the formation and down-cutting of channels that erode backwards from the downstream edge. As the experiment continues, the channels and their valleys extend into the uniform sloping surface by headward erosion, resulting in longer valleys, more branches and a greater dissection of the surface by those valleys.
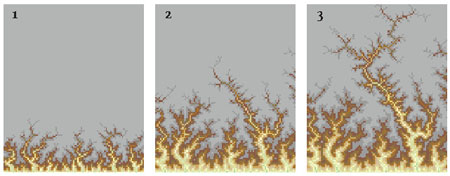
FIG 12. Model showing upstream erosion by tree-like (dendritic) river patterns. (Provided by Dimitri Lague from the work of A. Crave and P. Davy)
As we consider the various Regions and Areas of Southern England, we will summarise the present-day river patterns of each by simplifying the main directions of drainage involved. We will also give an impression of the present-day relative size of the more important rivers by quoting their mean flow rates as estimated in the National River Flow Archive, maintained by the Centre for Ecology and Hydrology at Wallingford.
It seems surprising that today’s often sleepy southern English rivers have been the dominant agent in carving the English landscape. However, even today’s rivers can become surprisingly violent in what are often described as hundred-or thousand-year floods. Floods in the past were certainly more violent at times than those of today, particularly towards the ends of cold episodes, when melting of ice and snow frequently produced floods that we would now regard as very exceptional.
THE ICE AGE TIMESCALE AND LANDSCAPE MODIFICATION
The most recent Ice Age began about 2 million years ago, and is still continuing in Arctic areas. At various times during this period ice has thickly covered most of northwest Europe. Recent research, particularly measurements of oxygen isotopes in polar icecaps and oceanic sediment drill cores, has revealed much of the detail of how the climate has changed during the current Ice Age. It has been discovered that long cold periods have alternated with short warm periods in a complex but rather regular rhythm. Looking at the last half-million years, this alternation has occurred about every 100,000 years, and this is now known to have been a response to regular changes in the way the Earth has rotated and moved in its orbit around the sun. A closer look at the last million years (Fig. 13) reveals that for more than 90 per cent of the time conditions have been colder than those of today. Warm (interglacial) periods, like our present one, have been unusual and short-lived, though they have often left distinctive deposits and organisms.
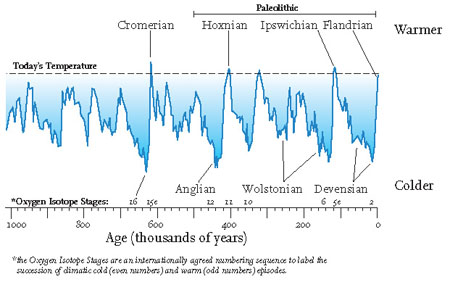
FIG 13. The last million years of global temperature change. *the Oxygen Isotope Stages are an internationally agreed numbering sequence to label the succession of climatic cold (even numbers) and warm (odd numbers) episodes.
One of the most important cold episodes (glacials), just under half a million years ago, resulted in the Anglian ice sheet. This was up to several hundreds of metres thick and extended from the north southwards, well into Southern England, covering much of East Anglia and the north London area (Fig. 14). As the ice spread slowly southwards, it was constricted between the Chalk hills of Lincolnshire and those of Norfolk. A wide valley, later to become the Wash and the Fens, was filled with ice to a depth well below that of present sea level. As the ice spread outwards from this valley it dumped the rock material it was carrying, including blocks and boulders up to hundreds of metres across, giving some idea of the tremendous power of the ice sheet. The direct evidence for the presence of an ice sheet is material in the surface blanket called till, or boulder clay (Fig. 15). This often rather chaotic mixture of fragments of rock of all sizes (large boulders mixed with sand and mud) lacks the sorting of the fragments by size that would have occurred in flowing water, and so must have been deposited from the melting of ice sheets.
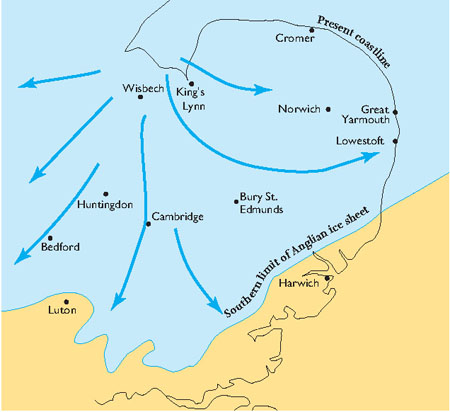
FIG 14. The Anglian ice sheet.
Much of the rest of the surface blanket that accumulated during the last 2 million years was deposited by the rivers that were draining the land or any ice sheets present. As ice sheets have advanced and retreated, so have the rivers changed in their size and in their capacity to carry debris and erode the landscape. Rivers have therefore been much larger in the past as melting winter snow and ice produced torrents of meltwater, laden with sediment, which scoured valleys or dumped large amounts of sediment. The gravel pits scattered along the river valleys and river terraces of Southern England, from which material is removed for building and engineering, are remnants of the beds of old fast-flowing rivers which carried gravel during the cold times.
There are no ice sheets present in the landscape of Figure 16. The scene is typical of most of the Ice Age history (the last 2 million years) of Southern England, in that the ice sheets lie further north. It is summer, snow and ice are lingering, and reindeer, wolves and woolly mammoths are roaming the swampy ground. The river is full of sand and gravel banks, dumped by the violent floods caused by springtime snow-melt. The ground shows ridges of gravel pushed up by freeze-thaw activity, an important process in scenery terms that we discuss below.
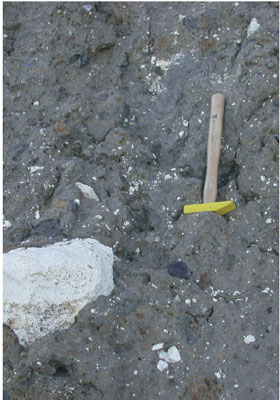
FIG 15. Boulder clay or till, West Runton, north Norfolk.
The present-day Arctic has much to tell us about conditions and processes in Southern England during the cold episodes of the Ice Age. Much of the present-day Arctic is ice-sheet-free, but is often characterised by permanently frozen ground (permafrost). When the ground becomes frozen all the cracks and spaces in the surface-blanket materials and uppermost bedrock become filled by ice, so that normal surface drainage cannot occur. In the summer, ice in the very uppermost material may melt and the landscape surface is likely to be wet and swampy. Ice expands on freezing, and so the continuous change between freezing and thawing conditions, both daily and seasonally, can cause the expansion of cracks and the movement of material, with corresponding movements in the surface of these landscapes. This movement can cause many problems in the present-day Arctic by disturbing the foundations of buildings and other structures.
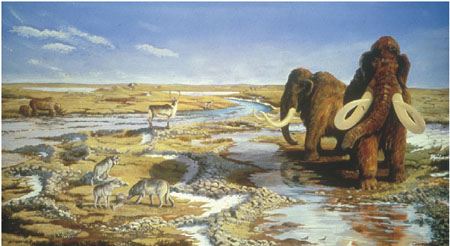
FIG 16. Artist’s impression of Southern England, south of the ice sheet, during the Ice Age. (Copyright Norfolk Museums and Archaeology Service & Nick Arber)
Remarkable polygonal patterns, ranging from centimetres to tens of metres across, are distinctive features of flat Arctic landscapes, resulting from volume changes in the surface blanket on freezing and thawing (Fig. 17). In cross-section the polygon cracks and ridges correspond to downward-narrowing wedges (often visible also in the walls of gravel pits in Southern England). Thaw lakes are also a feature of flat areas under conditions of Arctic frozen ground (Fig. 18). They appear to be linked to the formation of the polygonal features, but can amalgamate to become kilometres across and may periodically discharge their muddy soup of disturbed sediments down even very gentle slopes.