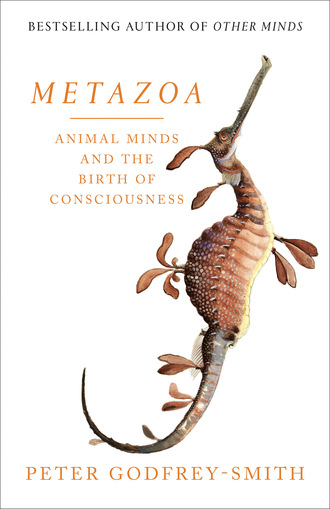
Полная версия
Metazoa
Animals can achieve some action without muscle. In comb jellies, the body is lined with bands of the hair-like cilia seen also in many single-celled organisms. The cilia are lined up vertically in what looks like a comb (hence the animal’s common name). Their motions enable the comb jelly, like its single-celled counterparts, to swim. (Comb jellies do also have muscle, which they use for steering.) Cilia are employed for small-scale motions in many other animals. But all the more extensive motions—the octocoral reach, the medusa’s swim, and other motions to come—are achieved through muscle.
In discussing the innovations that enabled animals to eventually play such an unusual role on Earth, I have been emphasizing new capacities on the side of action. Another animal feature, one I’ve not been talking much about in this chapter, is sensing. Sensing is always present, not just in animals but in all known cellular life. But some of the clues we have suggest that in these early stages in animal evolution, the conspicuous, unprecedented innovation was the creation of action on a new scale. This was the transforming factor.
Cnidarians today have a range of senses, and the same is true of likely ancestral animals at all historical stages. But cnidarians are “skinnier,” so to speak, on the sensory side than they are on the side of action. No eyes at all are found in corals or anemones, and only rather rudimentary eyes in most other cnidarians. (The big exception, box jellyfish, are thought to be a later product.) A polyp’s reach for food, a colony’s ascent and descent, and the firing of stinging cells are all tied to stimuli of various kinds, and cnidarians probably also invented a sense of balance, or a gravity sense. A medusa orients itself in the water by means of organs containing small crystals, called statocysts. The crystals are heavier than water, and when they move in response to a shift in the animal’s position, those motions can be detected. There may be other subtle forms of sensing, too, but in cnidarians, sensing is not what might be called their particular richness, their step into the unknown, the thing they do differently. Instead, the innovation was a new kind of action: large-scale, muscularly controlled motion.
With this transformation of animal life in our sights, let’s think for a moment about the mind-body problem in the background. Ordinary ways of thinking furnish us with a number of concepts that help us get a handle on what minds do. One is the concept of subjectivity. This concept arrives in a complementary pair with another: agency. Subjectivity is a matter of seeming, of for-me-ness. It points toward experience as something that happens to a person. Agency is a matter of doing, trying, initiating. Agency is by-me-ness; it is being a source of action and its effects. It points toward the things a person makes happen. Interestingly, the word “subject” (though not “subjectivity”) also has another set of connotations, in which a subject is a doer or initiator: subject as opposed to object. This is not the last time that these concepts will become entangled.
As everyday concepts, subjectivity and agency gesture toward different aspects of a person or animal, a more sensory side and a more active side. From an evolutionary point of view, however, these are closely tied together. Sensing has its raison d’être in the control of action. Nothing is gained biologically from taking in information that is not put to use. The evolution of the mind includes the coupled evolution of agency and subjectivity. But not everything has to develop in lockstep. There might be, at some stage, a breakthrough in the particular realm of action. A new kind of agency might come into being alongside simpler sensory capacities.
All through this part of the book, I am influenced by the thinking of the Dutch psychologist and philosopher Fred Keijzer, and his emphasis on the shaping of action as a central concern in the early evolution of nervous systems. The discussions in this chapter of the formation of action on a multicellular scale, the size and importance of that achievement, and its relationship to the animal body have all been influenced by him. In the area of the relations between sensing and action in very early animals, Keijzer has an interesting suggestion. He thinks that some new kinds of sensing may come “for free,” or almost for free, as a consequence of the shaping of complex action. Suppose you build an elaborate system for producing some sort of coordinated, choreographed motion. Often, to do this you will need some parts of the system to be sensitive to what other parts of the same system are up to. But then if something external affects the system, especially by touch, this event will automatically be registered to some extent, as it will disrupt the pattern of activity going on among those parts. The internal sensing within the system will—or could easily come to—register that something external has happened. Even if a nervous system were to be looking entirely inward (Keijzer is not suggesting that things are ever like that, but if it were), that system would be responsive to things on the outside. Such a system can’t help seeing outward, to some extent. New and expansive actions carry an expansion of sensitivity along with them.
The appearance of an asymmetry between complex action and simpler sensing at some early stage in animal evolution might be entirely an illusion. Complex sensing can be well hidden. But when thinking about the first forms of experience, or about what exists in animals before experience, it is interesting to imagine an animal whose motions outrun its senses, and ask whether, as Keijzer thinks, sensing would tend to automatically catch up.
Let’s step back from these speculations to the main themes emerging at this stage of the story. All living beings do things. They adjust their activities, and affect what is around them. But in animals, this took a new form. On the animal outgrowth of the tree of life, one thing produced was a multicellular self. In addition, the evolution of animals produced multicellular action, action achieved through sheets of cells that contract, twist, and grasp. Nerves and muscle made this possible; a sponge can do nothing like it. Action of this kind was a transformative invention in evolution; it changed everything.
It changed everything—eventually. When did this transformation begin, and what sort of animal set things rolling? Was it an animal that looked like a cnidarian, or something else, earlier? As we’ll now see, the engine of animal action, this engineer of Earth, had perhaps a fitful start.
Avalon to Nama
In the previous chapter we looked for clues about early forms of animal life, mostly by looking at animals alive now, but far from us. The outer limbs of the animal part of the tree of life, from our point of view, are very unclear. Things get clearer a few steps closer to us. Drawing evolutionary lines with time running up the page, the shape of some of the relationships looks like this:
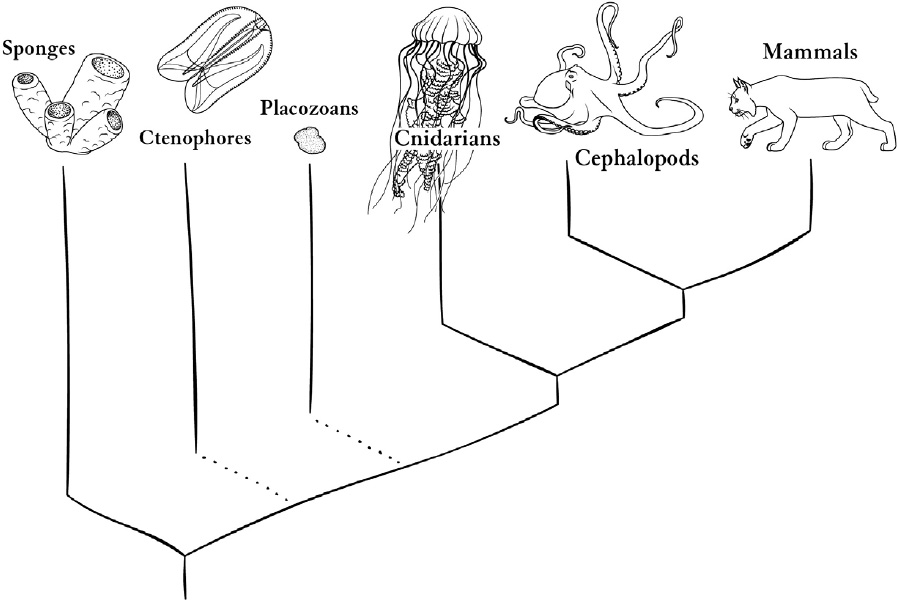
Nervous systems evolved somewhere below the split on the right that leads toward mammals and cephalopods on one side and cnidarians on the other. They may have evolved twice; this depends on unresolved questions about the tree, indicated by broken lines in the drawing.
All the branchings and evolutionary inventions discussed so far occurred before any fossil record of animals appears. The first period of time from which we have definite fossil evidence of animals is the Ediacaran, which began about 635 million years ago. The slow opening of the curtain on an animal fossil record reveals a scene very different from life around us now.
The setting is the seafloor, sometimes shallow, sometimes deeper, populated by various soft-bodied creatures, some tiny but some up to several feet across. Despite their soft bodies, a number of them left traces. Those traces show enigmatic forms—flower-like designs, whorls and discs, spirals and fractal branchings.
What reason is there to believe they were animals at all? In some cases this is indeed uncertain, and some remains may represent a wholly lost multicellular experiment, or experiments, far from the animals. But in at least some cases, they are animals. This was confirmed in 2018 by a student named Ilya Bobrovskiy, rappelling down a cliff in a remote part of Russia where unusually large and well-preserved remains of a famous Ediacaran organism, Dickinsonia, are found. As Bobrovskiy suspected, the rocks contained not just ordinary fossils, but remains that had been naturally mummified and preserved for over half a billion years. The mummified bodies contain cholesterol, a chemical made only by animals. Dickinsonia was flat, lived almost certainly on the seafloor, and looks like a bath mat, up to a meter long. It has no sign of eyes, limbs, or other familiar animal parts, and that is how it is with Ediacarans. They often have definite body forms—fronds and wheels, three-sided and five-sided—but no legs, fins, or claws, and no sign of complex senses like eyes.
No clear cases of cnidarians or sponges, the animals I’ve been discussing as clues, are known from the Ediacaran, either. But in each case there are possibilities. Some Ediacaran organisms look a fair bit like present-day “sea pens.” These well-named organisms are in the same group as the soft corals we visited at the start of this chapter. But rather than trees, each looks like an old plumed pen with its nib stuck in the seafloor and feathers spreading on a stem above.
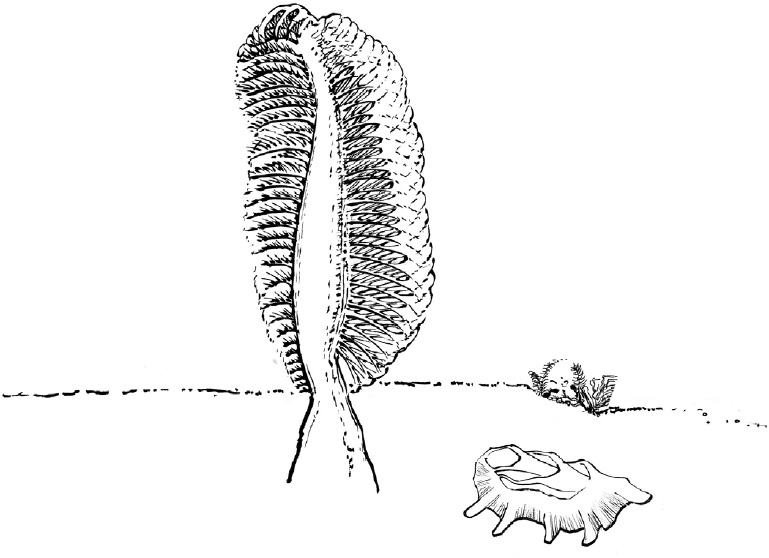
Whether any Ediacarans are closely related to sea pens remains controversial, as differences appear once one looks closely. Other Ediacaran organisms have branched fronds that also suggest cnidarian forms, but those similarities, too, may be misleading. A lot of Ediacarans were initially called “jellyfish,” by Reg Sprigg, who first uncovered Ediacaran fossils while inspecting disused mines in South Australia in 1946. Most of those fossils have now been interpreted differently, but up in the water were real jellyfish, most likely, their bodies scrunching into incomprehensibility when they fell.
The Ediacaran in the biological imagination has often seemed a quiet and placid time, a period with little interaction between organisms. There are almost no signs of predation—no half-eaten individuals, no sign of the built-in weapons, offensive and defensive, that animals tend to have now. There are no claws or spines. No signs are known of sexual specialization, either, though it is hard to tell, and no Ediacaran has ever been assigned to one sex or another. Sex was almost certainly present, though it probably coexisted with various kinds of asexual reproduction (as in cnidarians and sponges today). Densities were often high; one can see slabs of rock with a jumble of dozens or hundreds of organisms of several species. But even in these Hieronymous Bosch–like scenes, the animals are not obviously having much to do with each other. There may have been hidden interaction by means of lost soft parts, but much of the familiar machinery of contact between animals seems to be missing.
That placid image of the time is fine as far as it goes. Recent years, though, have seen the emergence of more detail, and the quiet Ediacaran has begun to resolve into something with a little more drama, certainly with transitions and changes.
As it looks now, there were three stages. These were distinguished by a young biologist, Ben Waggoner, about twenty years ago, and have stood up well as more data has come in. The stages have lovely names (thanks to Waggoner, with help from the geography). I say “stages,” but technically each is an “assemblage” (not as lovely a word); an assemblage is a collection of species as fossils, all laid down at roughly the same time.
The first of these assemblages is the Avalon, dating from about 575 million years ago. Even this first stage is fairly late in the Ediacaran as a whole. That period is bounded at its far end, about 635 million years ago, by the easing of an ice age, a massive glaciation that may have covered the whole Earth in ice. Conditions were quiet for a time, another ice age passed, and then the fossils appear soon after that. Oxygen levels may have increased significantly after this second ice age. Through all these early stages, however, one should imagine a low-oxygen world. This would have limited animal activity, to the extent that activity was even an option.
The Avalon assemblage, named after a place in Canada, features what seem to be stationary, plant-like, frond-ish organisms. (In a fortunate etymological confluence, “Avalon” in ancient Welsh means “the island of fruit trees.”) A lot of these creatures look superficially like a single large leaf, or a cluster of leaves, stuck in the seafloor. Inspected closely, each leaf is a sheaf of intricate branching elements.
The Avalon assemblage also has a possible sponge—conical, with the right sort of shape, though not reminiscent of any modern sponge group. Sponges in general are puzzling. Chemical evidence along with genetics suggests that sponges were present, even common, at this time, but fossils so far give us just this one conical candidate and one later possibility, recently discovered, that looks like an old downturned TV antenna, with thin rods coming out from its center.
The Avalon organisms seem to have lived in quite deep seas, too dark for photosynthesis, hundreds and perhaps thousands of meters down. That is today a sparsely populated and challenging zone, but it may have been a cradle for slow but innovative life back then. These creatures might have lived on tiny dissolved particles of organic carbon—their branching-upon-branching design has a “fractal” organization that maximizes surface area, allowing a steady uptake of this organic mist, along with oxygen to burn it.
Next came what looks like a shift. The White Sea assemblage, named for a location in Russia, dates from around 560 million years ago. These fossils show more diverse body plans. There are still no fins or legs, but in a few cases, the body plan and fossil traces strongly suggest that the animal could move.
The creatures of the White Sea assemblage lived on shallow seafloors, not the depths as in Avalon. These are seafloors that were, in a sense, alive. They are sometimes referred to as “microbial mats,” but Mary Droser of the University of California, Riverside, who has become a central figure in work on this stage, calls them “textured organic surfaces.” These surfaces contained more than bacteria and the like, probably including algae-like organisms and small embedded animals. The fossil record preserves the textures themselves—“wavy-crinkly lamination, elephant-skin textures …” A tangle of living and dead organisms at different scales made up a mostly two-dimensional surface, a flatland in the sea.
In this setting, new bodies and lifestyles are seen. There are still stationary organisms, raised up and sea-pen-like, but also a range of flatter forms, oriented to grazing on the mat. Some of these could move. Dickinsonia (with its mummified Russian cholesterol) seemed to graze in one place, then move on, leaving a series of faint whole-body footprints behind. Two others were more active, it seems. Kimberella is seen as a likely relative of molluscs. It looked like a crawling macaron, one able to scrape the mat’s surface with an extendable spade-like part.
There is also an enigma called Helminthoidichnites. This fossil, christened with that unbelievably difficult name (Helmin-thoid-ich-night-ies) in the nineteenth century, had initially been found in less ancient rocks, and interpreted as the tracks of a small burrowing animal—perhaps a worm or crustacean. Similar traces were eventually discovered in Ediacaran rocks, and have now been analyzed in detail by Mary Droser and Jim Gehling, in South Australia, near where the very first Ediacaran fossils were found.
This work has used a new kind of excavation, enabling the undersides of huge rock slabs to be studied as wholes. Scrutinized closely, some slabs show traces of complex patterns of movement. The living animal moved through different layers of the undersea mat, piling up levees as it went. The tracks home in on the bodies of other animals, including Dickinsonia. This is the first fossil evidence of scavenging, of an animal consuming the dead. It is also the first physical trace of targeted motion, movement aimed at a goal that is sensed. Initially the targets were dead bodies, but scavenging has natural transitions to predation, especially when the prey is stationary or slow.
I said that Helminthoidichnites was an enigma. All Ediacaran organisms are enigmatic to some degree, but in this case the enigma is extreme. For a long time, all we had was the trail, and no remains of the animal itself. Just as this book was being finalized, a candidate appeared, a tiny bean-shaped creature that might be the maker of the Helminthoidichnites traces. It was found in South Australia, the original Ediacaran home.
The period of the White Sea fossils, then, is marked by a shift: new bodies, new behavioral capacities, a different environment. A couple of other animals from this period also seem to have been mobile. Spriggina has a body with movement written over its form, superficially resembling a scurrying trilobite. There are no known traces of Spriggina movement, but that is not a surprise, as an animal must burrow or scrape in order to leave a mark. If you just glide over the top of the mat, no trace will come down to us, many millions of years later.
This was a time when oxygen levels continued to rise, slowly and erratically. Perhaps the sequence is something like this: with more oxygen, textured living surfaces developed. They became a resource for grazing, encouraging slow movement along the mat. Grazing leads to a concentration of resources in animal bodies, which then die. This makes the environment more patchy—there is lots of food here, less there. Motion becomes more valuable, as does the ability to follow scents in the sea, to track things down.
The third named stage, after Avalon and White Sea, is called “Nama,” after a site in Namibia, Africa. This is the youngest, leading up to the end of the Ediacaran. Given what had been going on just before, we might expect that the Nama phase would have more of this crawling complexity. But instead, these rocks are quieter. The crawling forms, surprisingly, are gone. The Helminthoidichnites traces are still there, and one interpretation of this period, called “Wormworld,” holds that a profusion of small tunnelers and burrowers were major players in this third stage. But those larger mobile animals that look at least dimly reminscent of molluscs and the like seem to have disappeared. Other than the burrowers, life in Nama moved back to swaying frond-like forms (though with fronds mostly different from before). No one knows why this happened. And the Nama assemblage seems to represent the stage leading up to the Ediacaran’s end.
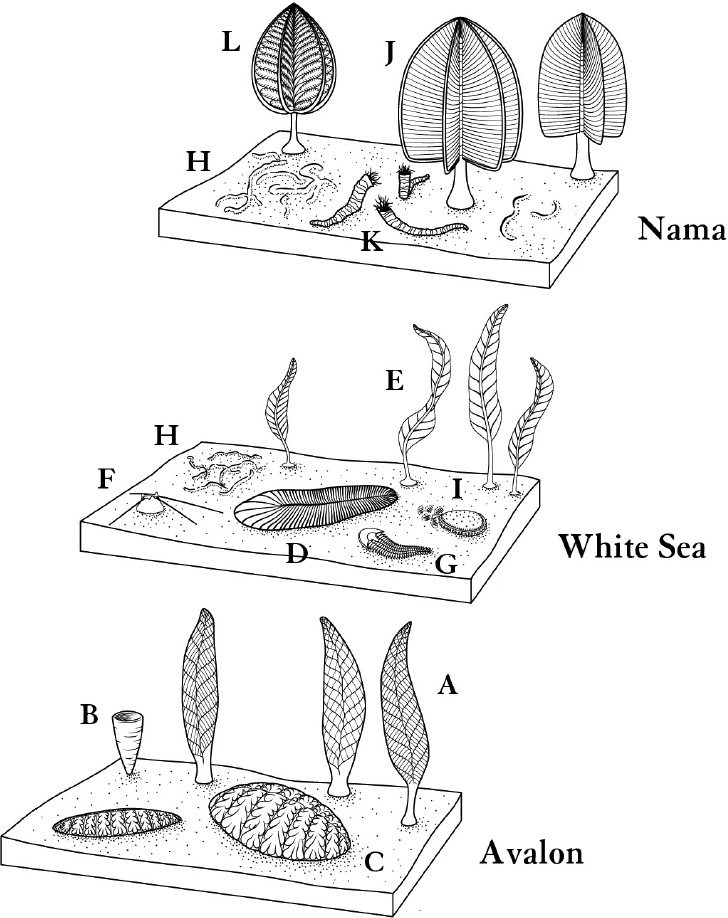
Three stages of the Ediacaran. Organisms include A: Charnia; B: Thectardis (a sponge?); C: Fractofusus; D: Dickinsonia; E: Arborea; F: Coroncollina (also a sponge?); G: Spriggina; H: Helminthoidichnites; I: Kimberella; J: Swarpuntia; K: Cloudina; and L: Rangea. Charnia and Rangea are some of the organisms that have been compared to sea pens.
How does this fit in with the themes in this chapter, with the attempt to discern clues about the evolution of animal action? We have a stage with stationary plant-like forms—Avalon, in the deeper sea—and then a transition to mobile creatures in shallower seas. Genetic evidence suggests that nervous systems evolved before any of these fossils were laid down, or perhaps during the first stage; the genetic dating is rough. Then there seem to be the beginnings of a regime in which new kinds of sensing and acting come online—White Sea. In Nama, this appears to fade.
If the Avalonians we know of were animals with nervous systems (not counting the possible sponge), what were they doing with them? It is tempting to say they might have been coordinating a reach or clasp, as in soft corals today. But in some cases where these organisms left unusually detailed fossils, no evidence of body openings has been found—unlike a soft coral, there is no mouth to bring food to. Instead, their whole body surfaces may have absorbed food—this, again, would make sense of a body design that maximizes surface area.
The frond-ish Avalonian organisms may not have been animals at all. Even if so, nervous systems probably did exist in some form before the crawling animals seen later in the Ediacaran. The evidence is good that nervous systems evolved in a body with something like a radial design. They might have been hidden in some of the flower-like bodies, but we might also need to look up.
I have been focusing on these seafloor forms because that is where fossil evidence was left. But all sorts of additional life may have been aloft in the water column—soft-bodied swimmers, akin to jellyfish and comb jellies. Some early stages in the evolution of nervous systems may have occurred up there. Filmy early swimmers will often be invisible to paleontology, as their delicate bodies are unlikely to fossilize into visible forms when they die. It has been suggested that early crawling animals might have evolved from a larva (an immature form) of a cnidarian-like animal, a larva that reaches the seafloor and starts to move along it. From there one can envision a sequence from mat-grazing and movement to behavior directed on other animals.
Discussions of the Ediacaran sometimes seem to show an understandable preference for making the story work using just the cast of characters present in fossils, and hence living on the seafloor. I often wonder whether, with respect to the evolution of behavior and interaction between animals, the scene on the ocean floor is just the tip of an iceberg, and a lot of what matters took place up in the water column, with animals that left little or no trace. If that is true, it’s hard to work out how the missing pieces of the puzzle might be filled in. But in evolutionary biology, it’s remarkable how often the apparently unknowable becomes knowable, as a result of a sudden technical advance, a mummified Dickinsonia, or a new theoretical idea.
This talk of a transition from swimming to crawling also brings up a stage that has gone unremarked upon so far, but a development we should look at closely. Peering back into the undersea fog of those times, one of the events that had enormous downstream consequences was the evolution of a new kind of body, called the bilaterian, or bilaterally symmetrical, body. These are bodies with a left-right axis, as well as a top and bottom. Our bodies are bilaterian bodies, along with those of ants, snails, and seahorses. We have arms and legs on each side, also eyes and ears; there is a left-right pairing of many of the body’s parts. Most animals now are bilaterians (that is true however you calculate “most”). So are some of the ancients—Kimberella, Spriggina, and others. Cnidarians like corals and jellyfish are not like this, and neither are comb jellies, sponges, or placozoans.
This body form evolved before the Ediacaran’s White Sea stage. It had to be as early as this, because some form of this design had to be in place before the branchings that sent different bilaterian animals down their various paths, and there are at least a handful of different bilaterians in the White Sea. The last common ancestor of you and a butterfly, also the last common ancestor of you and an octopus, lived at least this long ago.