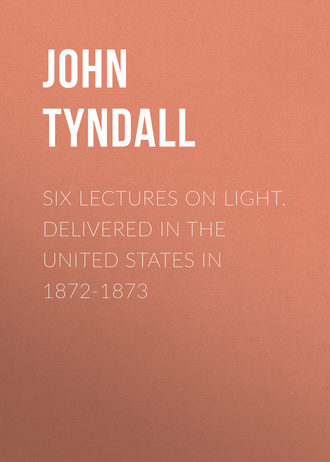
Полная версия
Six Lectures on Light. Delivered In The United States In 1872-1873
One step further for the sake of exactness. The light which falls upon a body is divided into two portions, one of which is reflected from the surface of the body; and this is of the same colour as the incident light. If the incident light be white, the superficially reflected light will also be white. Solar light, for example, reflected from the surface of even a black body, is white. The blackest camphine smoke in a dark room, through which a sunbeam passes from an aperture in the window-shutter, renders the track of the beam white, by the light scattered from the surfaces of the soot particles. The moon appears to us as if
'Clothed in white samite, mystic, wonderful;'
but were it covered with the blackest velvet it would still hang as a white orb in the heavens, shining upon our world substantially as it does now.
§ 8. Colours of Pigments as distinguished from Colours of Light
The second portion of the incident light enters the body, and upon its treatment there the colour of the body depends. And here a moment may properly be given to the analysis of the action of pigments upon light. They are composed of fine particles mixed with a vehicle; but how intimately soever the particles may be blended, they still remain particles, separated, it may be, by exceedingly minute distances, but still separated. To use the scientific phrase, they are not optically continuous. Now, wherever optical continuity is ruptured we have reflection of the incident light. It is the multitude of reflections at the limiting surfaces of the particles that prevents light from passing through snow, powdered glass, or common salt. The light here is exhausted in echoes, not extinguished by true absorption. It is the same kind of reflection that renders the thunder-cloud so impervious to light. Such a cloud is composed of particles of water, mixed with particles of air, both separately transparent, but practically opaque when thus mixed together.
In the case of pigments, then, the light is reflected at the limiting surfaces of the particles, but it is in part absorbed within the particles. The reflection is necessary to send the light back to the eye; the absorption is necessary to give the body its colour. The same remarks apply to flowers. The rose is red, in virtue, not of the light reflected from its surface, but of light which has entered its substance, which has been reflected from surfaces within, and which, in returning through the substance, has had its green extinguished. A similar process in the case of hard green leaves extinguishes the red, and sends green light from the body of the leaves to the eye.
All bodies, even the most transparent, are more or less absorbent of light. Take the case of water. A glass cell of clear water interposed in the track of our beam does not perceptibly change any one of the colours of the spectrum. Still absorption, though insensible, has here occurred, and to render it sensible we have only to increase the depth of the water through which the light passes. Instead of a cell an inch thick, let us take a layer, ten or fifteen feet thick: the colour of the water is then very evident. By augmenting the thickness we absorb more of the light, and by making the thickness very great we absorb the light altogether. Lampblack or pitch can do no more, and the only difference in this respect between them and water is that a very small depth in their case suffices to extinguish all the light. The difference between the highest known transparency and the highest known opacity is one of degree merely.
If, then, we render water sufficiently deep to quench all the light; and if from the interior of the water no light reaches the eye, we have the condition necessary to produce blackness. Looked properly down upon, there are portions of the Atlantic Ocean to which one would hardly ascribe a trace of colour: at the most a tint of dark indigo reaches the eye. The water, in fact, is practically black, and this is an indication both of its depth and purity. But the case is entirely changed when the ocean contains solid particles in a state of mechanical suspension, capable of sending the light impinging on them back to the eye.
Throw, for example, a white pebble, or a white dinner plate, into the blackest Atlantic water; as it sinks it becomes greener and greener, and, before it disappears, it reaches a vivid blue green. Break such a pebble, or plate, into fragments, these will behave like the unbroken mass: grind the pebble to powder, every particle will yield its modicum of green; and if the particles be so fine as to remain suspended in the water, the scattered light will be a uniform green. Hence the greenness of shoal water. You go to bed with the black water of the Atlantic around you. You rise in the morning, find it a vivid green, and correctly infer that you are crossing the Bank of Newfoundland. Such water is found charged with fine matter in a state of mechanical suspension. The light from the bottom may sometimes come into play, but it is not necessary. The subaqueous foam, generated by the screw or paddle-wheels of a steamer, also sends forth a vivid green. The foam here furnishes a reflecting surface, the water between the eye and it the absorbing medium.
Nothing can be more superb than the green of the Atlantic waves when the circumstances are favourable to the exhibition of the colour. As long as a wave remains unbroken no colour appears, but when the foam just doubles over the crest like an Alpine snow-cornice, under the cornice we often see a display of the most exquisite green. It is metallic in its brilliancy. The foam is first illuminated, and it scatters the light in all directions; the light which passes through the higher portion of the wave alone reaches the eye, and gives to that portion its matchless colour. The folding of the wave, producing, as it does, a series of longitudinal protuberances and furrows which act like cylindrical lenses, introduces variations in the intensity of the light, and materially enhances its beauty.
We are now prepared for the further consideration of a point already adverted to, and regarding which error long found currency. You will find it stated in many books that blue light and yellow light mixed together, produce green. But blue and yellow have been just proved to be complementary colours, producing white by their mixture. The mixture of blue and yellow pigments undoubtedly produces green, but the mixture of pigments is a totally different thing from the mixture of lights.
Helmholtz has revealed the cause of the green produced by a mixture of blue and yellow pigments. No natural colour is pure. A blue liquid, or a blue powder, permits not only the blue to pass through it, but a portion of the adjacent green. A yellow powder is transparent not only to the yellow light, but also in part to the adjacent green. Now, when blue and yellow are mixed together, the blue cuts off the yellow, the orange, and the red; the yellow, on the other hand, cuts off the violet, the indigo, and the blue. Green is the only colour to which both are transparent, and the consequence is that, when white light falls upon a mixture of yellow and blue powders, the green alone is sent back to the eye. You have already seen that the fine blue ammonia-sulphate of copper transmits a large portion of green, while cutting off all the less refrangible light. A yellow solution of picric acid also allows the green to pass, but quenches all the more refrangible light. What must occur when we send a beam through both liquids? The experimental answer to this question is now before you: the green band of the spectrum alone remains upon the screen.
The impurity of natural colours is strikingly illustrated by an observation recently communicated to me by Mr. Woodbury. On looking through a blue glass at green leaves in sunshine, he saw the superficially reflected light blue. The light, on the contrary, which came from the body of the leaves was crimson. On examination, I found that the glass employed in this observation transmitted both ends of the spectrum, the red as well as the blue, and that it quenched the middle. This furnished an easy explanation of the effect. In the delicate spring foliage the blue of the solar light is for the most part absorbed, and a light, mainly yellowish green, but containing a considerable quantity of red, escapes from the leaf to the eye. On looking at such foliage through the violet glass, the green and the yellow are stopped, and the red alone reaches the eye. Thus regarded, therefore, the leaves appear like faintly blushing roses, and present a very beautiful appearance. With the blue ammonia-sulphate of copper, which transmits no red, this effect is not obtained.
As the year advances the crimson gradually hardens to a coppery red; and in the dark green leaves of old ivy it is almost absent. Permitting a beam of white light to fall upon fresh leaves in a dark room, the sudden change from green to red, and from red back to green, when the violet glass is alternately introduced and withdrawn, is very surprising. Looked at through the same glass, the meadows in May appear of a warm purple. With a solution of permanganate of potash, which, while it quenches the centre of the spectrum, permits its ends to pass more freely than the violet glass, excellent effects are also obtained.7
This question of absorption, considered with reference to its molecular mechanism, is one of the most subtle and difficult in physics. We are not yet in a condition to grapple with it, but we shall be by-and-by. Meanwhile we may profitably glance back on the web of relations which these experiments reveal to us. We have, firstly, in solar light an agent of exceeding complexity, composed of innumerable constituents, refrangible in different degrees. We find, secondly, the atoms and molecules of bodies gifted with the power of sifting solar light in the most various ways, and producing by this sifting the colours observed in nature and art. To do this they must possess a molecular structure commensurate in complexity with that of light itself. Thirdly, we have the human eye and brain, so organized as to be able to take in and distinguish the multitude of impressions thus generated. The light, therefore, at starting is complex; to sift and select it as they do, natural bodies must be complex; while to take in the impressions thus generated, the human eye and brain, however we may simplify our conceptions of their action,8 must be highly complex.
Whence this triple complexity? If what are called material purposes were the only end to be served, a much simpler mechanism would be sufficient. But, instead of simplicity, we have prodigality of relation and adaptation—and this, apparently, for the sole purpose of enabling us to see things robed in the splendours of colour. Would it not seem that Nature harboured the intention of educating us for other enjoyments than those derivable from meat and drink? At all events, whatever Nature meant—and it would be mere presumption to dogmatize as to what she meant—we find ourselves here, as the upshot of her operations, endowed, not only with capacities to enjoy the materially useful, but endowed with others of indefinite scope and application, which deal alone with the beautiful and the true.
LECTURE II
ORIGIN OF PHYSICAL THEORIES
SCOPE OF THE IMAGINATION
NEWTON AND THE EMISSION THEORY
VERIFICATION OF PHYSICAL THEORIES
THE LUMINIFEROUS ETHER
WAVE THEORY OF LIGHT
THOMAS YOUNG
FRESNEL AND ARAGO
CONCEPTION OF WAVE-MOTION
INTERFERENCE OF WAVES
CONSTITUTION OF SOUND-WAVES
ANALOGIES OF SOUND AND LIGHT
ILLUSTRATIONS OF WAVE-MOTION
INTERFERENCE OF SOUND-WAVES
OPTICAL ILLUSTRATIONS
PITCH AND COLOUR
LENGTHS OF THE WAVES OF LIGHT AND RATES OF VIBRATION OF
THE ETHER-PARTICLES
INTERFERENCE OF LIGHT
PHENOMENA WHICH FIRST SUGGESTED THE UNDULATORY THEORY
BOYLE AND HOOKE
THE COLOURS OF THIN PLATES
THE SOAP-BUBBLE
NEWTON'S RINGS
THEORY OF 'FITS'
ITS EXPLANATION OF THE RINGS
OVER-THROW OF THE THEORY
DIFFRACTION OF LIGHT
COLOURS PRODUCED BY DIFFRACTION
COLOURS OF MOTHER-OF-PEARL.
§ 1. Origin and Scope of Physical Theories
We might vary and extend our experiments on Light indefinitely, and they certainly would prove us to possess a wonderful mastery over the phenomena. But the vesture of the agent only would thus be revealed, not the agent itself. The human mind, however, is so constituted that it can never rest satisfied with this outward view of natural things. Brightness and freshness take possession of the mind when it is crossed by the light of principles, showing the facts of Nature to be organically connected.
Let us, then, inquire what this thing is that we have been generating, reflecting, refracting and analyzing.
In doing this, we shall learn that the life of the experimental philosopher is twofold. He lives, in his vocation, a life of the senses, using his hands, eyes, and ears in his experiments: but such a question as that now before us carries him beyond the margin of the senses. He cannot consider, much less answer, the question, 'What is light?' without transporting himself to a world which underlies the sensible one, and out of which all optical phenomena spring. To realise this subsensible world the mind must possess a certain pictorial power. It must be able to form definite images of the things which that world contains; and to say that, if such or such a state of things exist in the subsensible world, then the phenomena of the sensible one must, of necessity, grow out of this state of things. Physical theories are thus formed, the truth of which is inferred from their power to explain the known and to predict the unknown.
This conception of physical theory implies, as you perceive, the exercise of the imagination—a word which seems to render many respectable people, both in the ranks of science and out of them, uncomfortable. That men in the ranks of science should feel thus is, I think, a proof that they have suffered themselves to be misled by the popular definition of a great faculty, instead of observing its operation in their own minds. Without imagination we cannot take a step beyond the bourne of the mere animal world, perhaps not even to the edge of this one. But, in speaking thus of imagination, I do not mean a riotous power which deals capriciously with facts, but a well-ordered and disciplined power, whose sole function is to form such conceptions as the intellect imperatively demands. Imagination, thus exercised, never really severs itself from the world of fact. This is the storehouse from which its materials are derived; and the magic of its art consists, not in creating things anew, but in so changing the magnitude, position, grouping, and other relations of sensible things, as to render them fit for the requirements of the intellect in the subsensible world.9
Descartes imagined space to be filled with something that transmitted light instantaneously. Firstly, because, in his experience, no measurable interval was known to exist between the appearance of a flash of light, however distant, and its effect upon consciousness; and secondly, because, as far as his experience went, no physical power is conveyed from place to place without a vehicle. But his imagination helped itself farther by illustrations drawn from the world of fact. 'When,' he says,' one walks in darkness with staff in hand, the moment the distant end of the staff strikes an obstacle the hand feels it. This explains what might otherwise be thought strange, that the light reaches us instantaneously from the sun. I wish thee to believe that light in the bodies that we call luminous is nothing more than a very brisk and violent motion, which, by means of the air and other transparent media, is conveyed to the eye, exactly as the shock through the walking-stick reaches the hand of a blind man. This is instantaneous, and would be so even if the intervening distance were greater than that between earth and heaven. It is therefore no more necessary that anything material should reach the eye from the luminous object, than that something should be sent from the ground to the hand of the blind man when he is conscious of the shock of his staff.' The celebrated Robert Hooke at first threw doubt upon this notion of Descartes, but he afterwards substantially espoused it. The belief in instantaneous transmission was destroyed by the discovery of Roemer referred to in our last lecture.
§ 2. The Emission Theory of Light
The case of Newton still more forcibly illustrates the position, that in forming physical theories we draw for our materials upon the world of fact. Before he began to deal with light, he was intimately acquainted with the laws of elastic collision, which all of you have seen more or less perfectly illustrated on a billiard-table. As regards the collision of sensible elastic masses, Newton knew the angle of incidence to be equal to the angle of reflection, and he also knew that experiment, as shown in our last lecture (fig. 3), had established the same law with regard to light. He thus found in his previous knowledge the material for theoretic images. He had only to change the magnitude of conceptions already in his mind to arrive at the Emission Theory of Light. Newton supposed light to consist of elastic particles of inconceivable minuteness, shot out with inconceivable rapidity by luminous bodies. Optical reflection certainly occurred as if light consisted of such particles, and this was Newton's justification for introducing them.
But this is not all. In another important particular, also, Newton's conceptions regarding the nature of light were influenced by his previous knowledge. He had been pondering over the phenomena of gravitation, and had made himself at home amid the operations of this universal power. Perhaps his mind at this time was too freshly and too deeply imbued with these notions to permit of his forming an unfettered judgment regarding the nature of light. Be that as it may, Newton saw in Refraction the result of an attractive force exerted on the light-particles. He carried his conception out with the most severe consistency. Dropping vertically downwards towards the earth's surface, the motion of a body is accelerated as it approaches the earth. Dropping downwards towards a horizontal surface—say from air on to glass or water—the velocity of the light-particles, when they came close to the surface, is, according to Newton, also accelerated. Approaching such a surface obliquely, he supposed the particles, when close to it, to be drawn down upon it, as a projectile is deflected by gravity to the surface of the earth. This deflection was, according to Newton, the refraction seen in our last lecture (fig. 4). Finally, it was supposed that differences of colour might be due to differences in the 'bigness' of the particles. This was the physical theory of light enunciated and defended by Newton; and you will observe that it simply consists in the transference of conceptions, born in the world of the senses, to a subsensible world.
But, though the region of physical theory lies thus behind the world of senses, the verifications of theory occur in that world. Laying the theoretic conception at the root of matters, we determine by deduction what are the phenomena which must of necessity grow out of this root. If the phenomena thus deduced agree with those of the actual world, it is a presumption in favour of the theory. If, as new classes of phenomena arise, they also are found to harmonise with theoretic deduction, the presumption becomes still stronger. If, finally, the theory confers prophetic vision upon the investigator, enabling him to predict the occurrence of phenomena which have never yet been seen, and if those predictions be found on trial to be rigidly correct, the persuasion of the truth of the theory becomes overpowering.
Thus working backwards from a limited number of phenomena, the human mind, by its own expansive force, reaches a conception which covers them all. There is no more wonderful performance of the intellect than this; but we can render no account of it. Like the scriptural gift of the Spirit, no man can tell whence it cometh. The passage from fact to principle is sometimes slow, sometimes rapid, and at all times a source of intellectual joy. When rapid, the pleasure is concentrated, and becomes a kind of ecstasy or intoxication. To any one who has experienced this pleasure, even in a moderate degree, the action of Archimedes when he quitted the bath, and ran naked, crying 'Eureka!' through the streets of Syracuse, becomes intelligible.
How, then, did it fare with the Emission Theory when the deductions from it were brought face to face with natural phenomena? Tested by experiment, it was found competent to explain many facts, and with transcendent ingenuity its author sought to make it account for all. He so far succeeded, that men so celebrated as Laplace and Malus, who lived till 1812, and Biot and Brewster, who lived till our own time, were found among his disciples.
§ 3. The Undulatory Theory of Light
Still, even at an early period of the existence of the Emission Theory, one or two great men were found espousing a different one. They furnish another illustration of the law that, in forming theories, the scientific imagination must draw its materials from the world of fact and experience. It was known long ago that sound is conveyed in waves or pulses through the air; and no sooner was this truth well housed in the mind than it became the basis of a theoretic conception. It was supposed that light, like sound, might also be the product of wave-motion. But what, in this case, could be the material forming the waves? For the waves of sound we have the air of our atmosphere; but the stretch of imagination which filled all space with a luminiferous ether trembling with the waves of light was so bold as to shock cautious minds. In one of my latest conversations with Sir David Brewster, he said to me that his chief objection to the undulatory theory of light was, that he could not think the Creator capable of so clumsy a contrivance as the filling of space with ether to produce light. This, I may say, is very dangerous ground, and the quarrel of science with Sir David, on this point as with many estimable persons on other points, is, that they profess to know too much about the mind of the Creator.
This conception of an ether was advocated, and successfully applied to various phenomena of optics, by the illustrious astronomer, Huyghens. He deduced from it the laws of reflection and refraction, and applied it to explain the double refraction of Iceland spar. The theory was espoused and defended by the celebrated mathematician, Euler. They were, however, opposed by Newton, whose authority at the time bore them down. Or shall we say it was authority merely? Not quite so. Newton's preponderance was in some degree due to the fact that, though Huyghens and Euler were right in the main, they did not possess sufficient data to prove themselves right. No human authority, however high, can maintain itself against the voice of Nature speaking through experiment. But the voice of Nature may be an uncertain voice, through the scantiness of data. This was the case at the period now referred to, and at such a period, by the authority of Newton, all antagonists were naturally overborne.
The march of mind is rhythmic, not uniform, and this great Emission Theory, which held its ground so long, resembled one of those circles which, according to your countryman Emerson, the intermittent force of genius periodically draws round the operations of the intellect, but which are eventually broken through by pressure from behind. In the year 1773 was born, at Milverton, in Somersetshire, a circle-breaker of this kind. He was educated for the profession of a physician, but was too strong to be tied down to professional routine. He devoted himself to the study of natural philosophy, and became in all its departments a master. He was also a master of letters. Languages, ancient and modern, were housed within his brain, and, to use the words of his epitaph, 'he first penetrated the obscurity which had veiled for ages the hieroglyphics of Egypt.' It fell to the lot of this man to discover facts in optics which Newton's theory was incompetent to explain, and his mind roamed in search of a sufficient theory. He had made himself acquainted with all the phenomena of wave-motion; with all the phenomena of sound; working successfully in this domain as an original discoverer. Thus informed and disciplined, he was prepared to detect any resemblance which might reveal itself between the phenomena of light and those of wave-motion. Such resemblances he did detect; and, spurred on by the discovery, he pursued his speculations and experiments, until he finally succeeded in placing on an immovable basis the Undulatory Theory of Light.