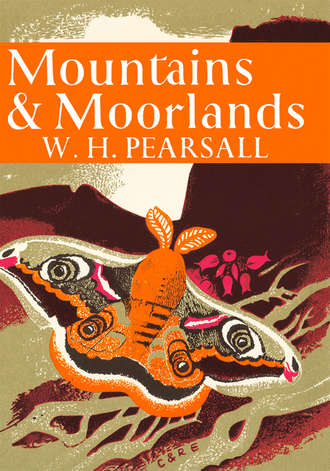
Полная версия
Mountains and Moorlands
Of course, where there are extensive areas of waterlogged soil and especially where peat is abundant, large amounts of ferrous salts may be present in solution in the soil-water. Wherever this becomes exposed to air it becomes oxidised either to metallic iron or to ferric salts and so considerable amounts of ferric substances may be precipitated. The orange-brown or metallic films due to this process are familiar objects round any peaty spring, and, long continued on a large scale, it has in the past been responsible for the production of deposits of bog iron ore. The same process continues in any peaty flush soil which receives drainage from waterlogged surfaces and into which air can, at times, penetrate. To the ecologist, the colours due to iron compounds are of great importance as visual evidence of the existence and progress of leaching or accumulation and also as useful clues to the presence or absence of aerated conditions in the soil. There are, of course, many chemical tests which can be used to confirm and extend these visible signs.
WATERLOGGED SOILS AND PEATS
What has just been said about waterlogged soils serves as a useful preface to the further consideration of this subject. These soils are distinct in being anaerobic or devoid of oxygen, and this is reflected in their “sad” appearance and in the characteristic blue-grey colours of the mineral matter due to ferrous iron salts. Such mineral soils are usually called gley soils, and, in the British uplands, they almost always possess another characteristic. The absence of oxygen prevents the decay of the organic matter derived from the plants growing on the surface. Consequently, upland waterlogged soils are also normally covered by layers of peat, and these are deep wherever waterlogging has been long continued, while they are usually shallower where this condition is of more recent origin. As a result of these accumulations of peat, the mineral soil below may be stained and mottled by peaty material.
The waterlogged peats are of two main types: (i) bog-peats and (ii) flush-peats. The bog-peats are widespread, covering the majority of stable upland soils and characteristic of those of slight slope (see Pl.s 22a and 24). They fall into two topographic types: those found on concave lowland forms, valley bottoms or lake basins, which have sometimes been distinguished as basin-peats, and, in contrast, those on long slopes and gentle ridges, for which Dr. H. Godwin coined the name blanket-bog, a term expressive of the way in which the peat covers all stable features of the original surface. Strictly speaking, basin peats are part of the blanket-bog in the uplands and it is only useful to separate them because they have, at times, a somewhat different and longer history as well as differences in present vegetation.
Flush-peats are also topographically conditioned, occurring only where water from a higher level impinges on the bog surface and brings to it a distinctive supply of minerals in solution. The three main types of dissolved materials (see here) give rise to the flush types: (a) lime-rich, (b) iron-rich, (c) peaty, but the first of these types is not nowadays common in our uplands, except where the bed-rock includes limestone. It is usually marked by the presence of certain mosses, and of gasteropod shells, elsewhere absent from the upland zone. Iron-rich flushes are, however, frequent, not only in the upper woodlands, but also around the bog margins. They are usually indicated by the presence of ferric-iron deposits, either on or in the surface layers of soil or peat. Moreover, if this peat is exposed to air-drying, the red-brown colour frequently becomes widespread. Certain types of vegetation (see here) are characteristic of these iron flushes. Peaty flushes are topographically distinct within the bog area but generally only show variants of the general bog vegetation.
While the properties of the soil in wet flushes are determined largely by the inflowing water in bog soils, certain other properties commonly exist to which attention may now be directed. The development of a peat-covering not only marks a stage in the soil development but it also modifies subsequent development by acting as a blanket which insulates, as it were, the mineral soil from the plants growing on the peat surface. At first these plants are rooted and are drawing mineral matter from the soil, but they get less and less dependent on it as time goes on and the peat gets deeper, and the soil water becomes more and more that derived from rain. As a general rule, then, the vegetation might be expected to show a transition in its mineral salt requirements from eutrophic, with high demands, to oligotrophic, with low salt requirements. Two things result from this: first, a succession of vegetation types, and secondly, a resultant succession of peat types. We shall see later that these facts help us in the analysis both of moorland vegetation and of the history of moorland areas. For the moment, however, we are more simply concerned with its effect on the properties of the soils. There will clearly be a change in composition throughout the peat profile, and the amount of mineral matter present in the peat will decrease as the level rises above the mineral base. This is apparently a general rule in upland peats, though, locally, flush effects may disturb the normal sequence. It should be noted, however, that it does not usually apply to the actual surface peats. Moor-burning is an almost universal practice nowadays, and its effect is to destroy the existing vegetation, leaving the mineral matter it contains to enrich the surface peat. Similarly, any form of oxidation of the surface peat, due, for example, to drainage, must have a similar effect, for the oxidation products of the organic matter are mainly carbon-dioxide and ash—the former of which escapes to the air, leaving the ash to increase the amount of mineral matter in the residual peat. Thus the surface peat, where moor-burning is practised, commonly contains more ash than the layers below it. The table opposite gives illustrative figures from peat-profiles in different British areas.
There are, of course, other effects which appear to be associated with this distribution of ash. Thus the acidity of the peat almost always increases from the lowest levels upwards—showing a general correlation with the decreasing ash content.
It will be seen from Table 5, and it follows from the arguments used above, that typical upland peats are remarkable for the small amount of ash they contain, and when we seek to define the term bog, it is usual to regard it as referring to peat of this type supporting an extremely oligotrophic vegetation. In this use of the term a bog is mainly dependent on atmospheric water (i.e. rain) and uninfluenced by ground-water. The term bog contrasts in usage with the term fen—derived from the extensive peat deposits in East Anglia. In terms of this usage, fen-peat is characterised by its high mineral content and hence by its dependence on ground water. It usually shows signs of an abundance of lime and is always lime-saturated peat with a luxuriant and eutrophic vegetation of tall reeds and small trees, willows and alders. Peats of this type are almost non-existent in the British uplands to-day, although long ago they existed in some of the hollows where lime-rich waters accumulated in small ponds and lakes. These now often show their former character by an underlying bed of marl. Almost all these areas are now deeply buried beneath bog-peat, and only small areas of flush peat remain round the bog margins to illustrate the effects of this type of peat on the vegetation. Where the basal peats were originally calcareous the succession of peat types above usually shows a much more gradual decrease in mineral content, and the sequence of vegetation types was often different.
Table 5 ASH CONTENTS (AS PER CENT OF THE DRY WEIGHT) OF PEAT SAMPLES AT DIFFERENT DEPTHS

Finally, where a peat-profile generally shows signs of differences in botanical composition at different levels, it also usually shows differences in physical structure. The changes are due partly to alterations in the composition of the vegetation from which the peat was formed, and sometimes they may be due to changes in the conditions (of humidity or temperature or drainage perhaps) under which the peat was formed. As a general rule, however, the most important progressive change in the peat must be associated with its decomposition as it gets older. Two sorts of change are possible: partial oxidation, which occurs particularly in the surface layers, and the slower changes which can ensue in water-saturated peat from which oxygen is absent. We assume that these are mainly hydrolytic—that is, caused by the slow action of water on the organic materials present. These are the changes which are generally implied when we say that a peat is humified or that it is undergoing humification. They are thought to result in the plant-remains gradually becoming gelatinous, so that, although the peat appears to retain visible structure, it escapes as a jelly through the fingers when squeezed in the hand. In contrast, the more recent peats usually retain a firm and fibrous structure. Even when a peat bed appears, on first opening it up, to consist of more or less uniform material, the bottom layers will normally differ in the degree of humification from those at the top. One result of this is that if such a bed is cut and a profile exposed to the air, it will soon appear to consist of two different types of peat. The fibres quickly become prominent and some are exposed by weathering, while the upper layers which contain them are often more quickly oxidised and so may become darker in colour. The lower layers, however, remain as a damp gelatinous mass and show less alteration on exposure. After a period of exposure they may thus appear to be of quite different composition, although the apparent difference is really one mainly of different physical and chemical condition.
THE BIOLOGICAL CHARACTERS OF SOILS
The peaty nature of upland soils is one of their most easily observed attributes. It is by no means confined to waterlogged areas, but it is equally noticeable on leached podsolic soils and even in the early stages of soil-formation on mountain-top detritus. This clearly indicates that the climatic effects characteristic of these three extreme types of habitat—waterlogging, leaching and low temperatures—are alike in leading to humus or peat accumulation in the soils affected. They do so in a generally similar way by reducing the activity of the soil micro-organisms.
Their effects differ in some respects and it will therefore be convenient to consider them separately, although actually they overlap in nature to a considerable degree. The effects produced on and by the soil organisms in their turn affect the vegetation of larger plants, and hence, as later chapters will show, have much effect on the larger animals.
Numerically, the soil flora mainly consists of vast numbers of bacteria and fungi. These are colourless plants, mostly of microscopic size in the soil, that obtain their nutriment by chemically changing the remains of dead plants and animals. They are responsible for what we usually call decay, although the chemical processes involved are analogous to and, indeed, often identical with, the processes of food digestion and utilisation in animals. The breakdown of plant organic matter in soil is, however, very generally initiated by small invertebrate animals, sometimes by the larvae of flies but particularly, as Charles Darwin showed, by earthworms. These break down the cellular structure of plant-remains and partly transform it, making it suitable for further transformation by fungi and bacteria. Most soils also contain single-celled animals, protozoa, which browse on the fungi and probably serve to keep them in check. There are also insects, such as springtails and fly larvae, whose role in soil economy is usually less well known.
This large soil-population requires air, or rather oxygen from the air, for breathing and it is consequently said to be aerobic in character.
In a normal soil the chemical materials produced during these transformations by the soil organisms are substances containing oxygen, carbon dioxide, which escapes to the air, nitrates, which contain the nitrogen which was present in the animal and plant proteins, and other substances such as sulphates and phosphates. Of these, nitrates are generally regarded as most important because they are quantitatively the materials a normal plant requires from the soil in largest amounts. Thus the fertility of a soil is usually determined very largely by the rate at which it can produce nitrates, i.e. the rate at which the nitrogen locked up in the decaying organic materials can be released in a form available to plants. In actual fact, plants can also use ammonia, but the amounts of ammonia in a natural soil are normally small. This substance is the first simple substance to be formed by bacteria and fungi during the soil decompositions. It is converted by other soil organisms, the nitrifying bacteria, into nitrate. These processes of ammonia production and nitrate formation seem to be particularly sensitive to adverse soil conditions. So also are the parallel processes of nitrogen fixation by which a fertile soil is usually able to increase its nitrogen content (to an extent which balances leaching losses) by fixing the gaseous nitrogen present in the air. This process is brought about in most soils by bacteria, as well as by the nodule-forming organisms which are found living in the root-nodules of leguminous plants like peas, beans and clover. Adverse soil conditions almost always produce their effects by retarding these processes as well as the numerous other processes, e.g. of decomposition, going on in the soil. Special effects are produced by the different adverse factors.
AEROBIC AND ANAEROBIC—OXIDISING AND REDUCING SOILS
The principal result of a soil becoming saturated with water is that the amount of oxygen in the soil is reduced to vanishing point. Consequently as most of the soil organisms are aerobic, requiring oxygen, the soil population is reduced to the minimum, and there can remain active only a few anaerobic organisms with specialised methods of maintaining their existence without oxygen. The products of the decompositions going on in the soil also change in character. Instead of the formation of carbon dioxide, nitrates, sulphates and phosphates, all containing oxygen, there may be produced instead marsh-gas (or methane, CH4), ammonia (NH3), sulphuretted hydrogen (H2S) or other sulphides, and sometimes phosphine (PH3), a series of compounds devoid of oxygen. All of these products are associated with the activities of anaerobic types of moulds or bacteria, the latter usually being most abundant. The microflora of waterlogged soils is thus specialised in character as well as poor in numbers, while the products of anaerobic composition include substances, in addition to those mentioned above, which may be toxic to the larger rooted plants. Some of the products are also responsible for other manifestations peculiar to boggy soils, such as the “will o’ the wisp” and “corpse-light,” these being attributed to the burning of the highly inflammable marsh and phosphine gases.
In effect, in contrasting waterlogged and aerated soils in this way, we are contrasting two sorts of micro-biological activity—oxidising and reducing—depending on whether the organisms can form chemically oxidised products like carbon dioxide and nitrates or chemically reduced substances like marsh-gas and ammonia.
The particular value of being able to recognise these possibilities is because they give us information as to the effect of the soil conditions on the action of living organisms, and we may infer that the conditions which affect the soil flora will also affect its fauna (see here) as well as the larger plants. Moreover, the level of oxygen content which produces these biological effects is low and it is not one which can be detected in the field with any certainty, if at all, by measurements of soil oxygen. For our present purpose, therefore, it is useful to think of upland soils as belonging to the two types named above.
oxidising soils contain reducing soils contain nitrate ammonia carbon dioxide marsh-gas (methane) sulphate sulphides phosphate phosphine ferric-iron ferrous-ironIt is useful also to realise that a large proportion of wet soils may be oxidising in drier periods and reducing in wet.
MULL AND MOR
In some respects the quantitative effects of leaching are similar to those produced by saturation with water—namely, a great reduction in the activity of the soil organisms. The qualitative effects of leaching on the soil micro-flora are, however, even more pronounced, and so much so that it is customary to give a special name, mor, to the peaty humus formed in leached soils, in order to distinguish it from the more fertile leaf-mould (or mull) typically associated with fertile forest soils. While mor is chemically different, as we shall see later, its most noticeable distinguishing features are biological and are easily recognised. There is a vegetation dominated by plants such as heathers, bilberry and wavy hair-grass (Deschampsia flexuosa), and normally an absence of earthworms. Usually, too, no tree seedlings are to be found except those of pine and birch. Moreover, leguminous plants such as clover are absent, while suitable tests show that the soil lacks nitrogen-fixing bacteria or, at least, effective strains of this type. Finally, the mor soil has a high and characteristic degree of acidity (see here), normally marked by a pH value below 3·8. It yields low proportions of ammonia, while nitrates are absent. It is evident, indeed, that in mor the rate and extent of nitrogen transformation is greatly reduced, presumably by the reduction in the numbers of suitable bacteria. It is in fact usually considered that the micro-flora of this type of humus contains few bacteria and is mainly one of moulds and of other fungi like actino-mycetes and basidiomycetes, but the evidence is far from conclusive owing to the difficulties of identifying these microscopic organisms in a dark-coloured peaty soil. Moulds and other fungi are generally more tolerant of acidity than are many soil bacteria, which fail to develop either in acid or in lime-deficient maedia.
It may be useful to add here a note on the soil animals which are more characteristic of mor soils. They include certain mites, the larval stages of two-winged flies (Diptera), click-beetles (Elaterideae), and often centipedes and predatory beetles. In contrast, earthworms, snails and millipedes are particularly characteristic of good forest leaf-moulds.
Mor soils in the strict sense in Britain are confined to the acid types of soil (normally with a podsol profile) distinguished in an earlier section. Characteristic mull soils are found on lime-deficient as well as on lime-saturated soils. More strongly base-deficient soils generally have humus of this type which produces nitrates (for example) rather slowly and may, at times, be somewhat intermediate in other respects.
The third factor which must profoundly affect the properties of the soil organic matter is the low temperature of many upland habitats. There can be no doubt that the soil organisms, like other forms of life, have their activity greatly reduced by low temperature. Hence the rate of decomposition of soil organic matter declines very rapidly in cold climates and peat accumulates, for the rate at which higher and larger plants form organic matter is less affected by low temperature, depending rather on the carbon-dioxide content of the air and on light. So far as I am aware, little or no investigation of the characters of this peaty material has been attempted, and the matter would probably be most easily examined by studying the rather peaty humus that accumulates on and among the mountain-top detritus. This is usually a black and easily crumbling peat generally containing a good deal of sand. It differs very markedly from a typical mor, such as that from below heather, for example, which is generally red-brown in colour, closer in texture and more acid. On the other hand, both soil-types have certain features in common such as some resemblance in fauna, e.g. scarcity of worms and frequency of dipterous larvae. There is also a good deal of evidence, though it is mainly derived from studies in arctic regions, that the bacterial processes affecting the accumulation of nitrogen (by fixation) and its liberation in forms suitable for plant food are especially curtailed by low temperature. As a result, there is certainly a notable scarcity of soluble forms of nitrogen in mountain-top habitats, though the limited available evidence suggests that this scarcity is not as severe as is the case in either bog-peat or acid mor. In my own observations, about half the tested soils have given traces of nitrates in late summer, and higher proportions might possibly be observed at other times. The interesting feature of the samples of humus from among mountain-top detritus has been their high proportion of nitrogen, usually 4 per cent of the total humus content. This does in fact suggest that there has been a rather slow decomposition of nitrogenous materials, though this is not the only possible explanation. The following short table summaries a few values for the nitrogen proportions of characteristic humus types.
Table 6 NITROGEN CONTENT OF DIFFERENT SOIL TYPES AS PER CENT OF THEIR HUMUS CONTENTS
Soil Vegetation Percentage Mountain-top detritus (2800–3000 ft.) Moss-lichen grassland (see p. 85) 3·6–4·0 Mor over podsol (700–1000 ft.) Heather-moor 1·0–2·0 Upland bog peat (1200–1500 ft.) Mixed-bog with bog-moss (p. 147–152) 1·7–2·4 Flushed brown earth with mull Oak-wood with soft-grass 3·1–3·5*This table brings out the low nitrogen content of the upland bog-peats and mor when contrasted with the mull humus of an oak-wood. Still more evident would this contrast be if we included the humus of lowland woods or of agricultural soils. The well-decomposed humus of a fertile arable soil, such as a wheat field, has commonly a nitrogen content of between 4·5 and 5 per cent, a value which seems to be a characteristic of soils in the lowland north temperate climate.
SIGNIFICANCE OF NITROGEN CONTENT OF HUMUS
The lower nitrogen content which is characteristic of upland peats and humus is due partly to the slow breakdown of the original plant materials. These, as a general rule, are rich in carbon and poor in nitrogen, though these proportions vary with the plant species. Consequently undecayed plant remains reflect principally the low nitrogen content of the original material. On the other hand, the ultimate nature of the humus produced depends also on the relative rates of breakdown and removal of the two main components—which are the carbonaceous and nitrogenous compounds. In many peats the soil bacteria are unable to attack cellulose, one of the principal materials containing carbon—a substance which makes up the bulk of plant cell-walls and skeletal structures. Slow though the breakdown of nitrogenous matters may be, therefore, it still is often more rapid than that of the chief carbon compounds, so that the latter gradually become more abundant and the percentage of nitrogen remaining in the humus becomes less. This condition has a further harmful effect on the activity of the soil fungi and bacteria, for most of these organisms prefer a growth medium containing a low C/N ratio. Thus in order to facilitate the breakdown of dead leaves in a rubbish-heap, gardeners often mix with it nitrogenous manurial materials, a treatment that results in a greatly accelerated rate of bacterial decomposition of the plant remains.