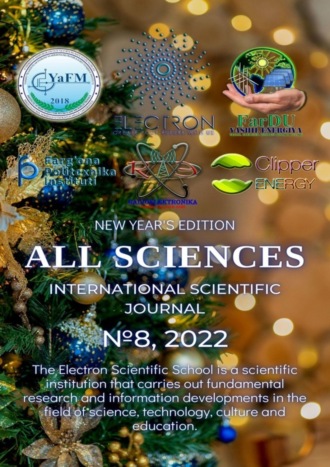
Полная версия
All sciences. №8, 2022. International Scientific Journal
Laser processing of thin films deposited on a substrate is used to form film elements widely used in instrumentation and microelectronics. Laser processing of thin films is characterized by high accuracy and locality, non-contact, good controllability and in most cases sufficient performance. In the modern technology of semiconductor devices, methods of processing materials using ionizing radiation are becoming increasingly important [1]. Special attention is paid to the modification of the properties of layers under the action of short laser pulses, when, along with ordinary thermal heating of films, the influence of factors having a non-thermal nature is possible.
The physical mechanisms of action of laser radiation on thin films are in many ways similar to the effect of radiation on massive materials, but they have some features.
This paper presents the results of studies of the effect of g-radiation and laser irradiation on the kinetic coefficients of polycrystalline films of narrow-band semiconductors. The objects of research were polycrystalline layers of lead and bismuth chalcogenides and their compounds obtained by thermal vacuum condensation under various technological conditions. The substrates were quartz, polyimide (PM-1) and mica. The thickness of the obtained films was 0.34 microns. The films were irradiated with Co60 g-quanta and an industrial GIG-1M laser (D=15J, tim =50 ns) in air and in vacuum. Measurements of electrical conductivity, Hall coefficient and thermal EMF were carried out.
Electron microscopic studies have shown that with an increase in the condensation temperature, the size of the crystallites increases. When exposed to laser pulses of Pb0.8Sn0 films.2Te condensed at a temperature Tc = 373 K, it was found that at irradiation energies W > 0.15 J /cm2, there is a violation of the adhesion of the condensate with the substrate. In this regard, the irradiation energies were less than the specified value.
It should be noted that these energies are also less than the energy of the calculated value of the peak melting energy of films during laser processing in the nanosecond range, which is 02J/cm2 [1].
This paper presents studies of the effect of laser annealing (LO) on the kinetic properties of polycrystalline Pb0.8Sn0.2Te films obtained on polyimide and mica substrates by thermal vacuum technology at various condensation temperatures [2]. Irradiation was carried out in the modulated Q-factor mode with an industrial laser with a ruby emitter (l = 0.69 microns, tim = 50 ns). The energy density in the laser pulse was adjusted by focusing the light beam. The kinetic coefficients of the films were measured as a function of the number of laser pulse effects. At the same time, the structure of the initial and irradiated films was studied using scanning electron microscopy.
The conductivity s, the concentration of pH carriers and the coefficient of thermal emf a were measured depending on the number of laser pulses of films condensed at different substrate temperatures. The results of the conducted studies have shown that with an increase in Tc, the conductivity of films s increases, and the coefficient of thermal emf a decreases. When exposed to laser pulses, a decrease in s and a is observed in the films.
Electron microscopic studies of the films have shown that with an increase in Tc from 300K to 600K, the size of the crystallites increases from (5-6) 102 to 104 oA, and in these films with an increase in Tc, an increase in s and a decrease in a are observed.
Noticeable structural changes in LO were observed in films obtained on mica at Tc = 570K, i.e. at higher condensation temperatures. Here, the growth of single-crystal fragments is detected, the size of which was many times larger than the size of the crystallites in the original non-irradiated condensates.
The processes responsible for crystallization phenomena, in our opinion, are partial melting of condensates under laser irradiation (partial, since the energy in the pulse is less than the melting threshold) and shock crystallization (accelerated crystallization in the solid phase).
The nature of the change in the concentration of holes in Vi2SbxTe3 films at g-irradiation (Co60 source, intensity 103P/s) in freshly deposited films and a film pre-annealed in air at 420 K for 3 hours, in which the concentration of holes before annealing coincided with the initial concentration of holes, is given.
The following patterns were noted:
1. In samples with initial values of the concentration of holes p ~ 8 1018 cm-3, g – irradiation leads to their monotonous increase with an output at Fd > 108P for saturation (curve 1); at 1019
2. At p> 5x1019 cm-3, with an increase in Fd, a decrease in concentration is observed with a decrease in the intensity of the process as the Fd increases (curve 3); in the same films, after thermal annealing, leading to a decrease in concentration, the process of decreasing concentration with an increase in Fd also slows down.
To explain the observed phenomena, it is necessary to accept the following. In technological regimes that provide a high concentration of holes, along with anti-structural defects, tellurium vacancies are formed in films [3]. Under g-irradiation, two processes are responsible for changes in the concentration of holes:
a) radiation-stimulated diffusion of antistructural atoms along vacancies with the displacement of the latter to the effluents – the boundaries of crystallites and dislocations;
b) displacement of tellurium atoms into internodes.
The intensity of the first process is proportional to the concentration of vacancies and is more energetically advantageous relative to the second process. The first process is accompanied by a decrease in the number of acceptors, and the second by an increase, therefore, depending on the initial concentration of tellurium vacancies in films, two types of changes in the concentration of holes at g – irradiation are possible, which is observed experimentally. The output of the p (Fd) dependence on saturation corresponds to the establishment of equilibrium in the course of both processes.
List of used literature
1. K.E.Anarkulov, M.M.Akhmedov, D.A.Yusupova, R. T. Rasulov, B. Duliev Kinetic processes in thin films of bismuth and lead chalcogenides under the influence of g- and laser irradiation. Uzbek Physical Journal, V. 4, No.2, 2002, pp. 113-116
2. S.H.Shamirzaev, D.A.Yusupova. Investigation of the electrophysical properties of polycrystalline films of bismuth-antimony tellurides containing nanogranule Khozirgi zamon fizikasining dolzarb muammolari. Republic of ilmiy konferentsiyasi materialari typlami Termiz 1- May, 2013 y.45-46 b.
3. D.A.Yusupova. Study of electrophysical properties of nanocrystalline films Bi2Te3 – Sb2Te3. «Integration of Sciences» International scientific and Practical journal. Moscow Issue No. 4 (19) (June, 2018), from 52—54
THE FIRST STAGE OF ACCELERATOR TECHNOLOGY DEVELOPMENT
UDC 29.01.09
Abdurakhmonov Sultonali Mukaramovich
Candidate of Physical and Mathematical Sciences, Associate Professor of the Faculty of Computer Design Systems of the Fergana Polytechnic Institute
Ferghana Polytechnic Institute, Ferghana, Uzbekistan
Аннотация. История ускорительной техники берёт своё начало ещё во времена самых первых исследований в области изучения строения вещества, и, хотя вопрос о строении материи был поставлен ещё в глубокой древности, его активное развитие начинается лишь чуть ранее открытия радиоактивности Анри Беккерелем. Самые первые попытки в области увеличения энергии генерируемых частиц были приложены ещё во времена первых трубок Крукса, в которых обеспечивался высокий вакуум, что позволяло обеспечить вылет приличного потока электронов под действием термоэлектронной эмиссии.
Ключевые слова: история, ускорители заряженных частиц, линейные ускорители, циклотроны, опыты Резерфорда.
Annotation. The history of accelerator technology dates back to the time of the very first research in the field of studying the structure of matter, and although the question of the structure of matter was raised in ancient times, its active development begins only a little earlier than the discovery of radioactivity by Henri Becquerel. The very first attempts in the field of increasing the energy of the generated particles were made back in the days of the first Crookes tubes, in which a high vacuum was provided, which made it possible to ensure the departure of a decent flow of electrons under the influence of thermoelectronic emission.
Keywords: history, charged particle accelerators, linear accelerators, cyclotrons, Rutherford experiments.
But if we proceed from the very beginning, then in the history of accelerators we can find many outstanding inventions, new and bright physical ideas, in some cases, having the character of a scientific discovery. However, the development of methods for accelerating charged particles and the pursuit of higher energies has never been an end in itself and necessarily obeyed mainly the logic of the development of nuclear physics and the resulting high-energy physics.
Previously conducted research and construction in the field of accelerator physics can be depicted using a diagram, so the existence of objective laws of the development of accelerator technology is simply and clearly convinced by such a dependence on the time of the maximum energy achieved in laboratory conditions. On a logarithmic scale, this dependence is reflected by a straight line, which, with some reservations, both existing installations and projected machines fall into. That is, the energy of artificially accelerated elementary particles increases exponentially by an order of magnitude every seven to eight years, which reflects the objective regularity of the development of science and high-energy physics. With all the importance of new ideas in accelerator physics, it should not be noted that their appearance did not cause noticeable fractures on this line and did not lead to such a case, the presence of any obvious deviations.
Probably, the first considerations about obtaining artificially accelerated particles appeared together with the birth of experimental nuclear physics after the historical experiments of E. Rutherford in 1919, although by that time there were already high-voltage X-ray tubes and installations for producing "channel rays", to a certain extent, deserving the name accelerators. The capabilities of high-voltage technology of that time, and the energy of alpha particles of natural radioactive isotopes, with which the accelerators were designed to compete, determined the immediate goal – to obtain particles with an energy of the order of several MeV. However, of course, the fundamental advantages of accelerators were also clear – the possibility of accelerating protons and other elementary particles, as well as the directivity and high intensity of the beam, equivalent to tens and hundreds of kilograms of natural radioactive preparations. Interestingly, in the 20s, quite a lot of ideas of acceleration to high energy were expressed, which were ahead of their time and embodied in specific installations only after many years.
Nevertheless, the first artificial nuclear reaction – the splitting of the lithium nucleus by protons with an energy of 700 keV – was carried out by the staff of Rutherford J. By Cockcroft and E. Watson in 1931 and immediately repeated in several laboratories. This date can be considered the beginning of the history of accelerators.
The Cockcroft-Walton installation consisted of two main elements – a high voltage generator and an accelerator tube. Both of them technically underwent significant modifications in the future. One of the main stages in the development of electrostatic accelerators was the invention in 1929 by R. Van de Graaf from Preston University in the USA of a high voltage generator with mechanical charge transfer. The increase in energy in these machines was restrained mainly by the electrical strength of the support insulators and the accelerator tube, but the use of forced potential distribution soon allowed to obtain an energy of 2.5 MeV. In the USSR, in 1938, an electrostatic accelerator at 3.6 MeV was launched in Kharkov. It is also important to note that by the end of the 50s, the accelerator tube of a serial electrostatic accelerator could withstand an order of magnitude more, namely 16 MV.
Nevertheless, the limited possibilities of the electrostatic acceleration method were obvious, and the development of nuclear physics urgently required a transition to energies of the order of ten MeV, comparable to the average binding energy of a nucleon in the nucleus. Therefore, the emergence of resonant methods that do not require high voltages should be considered a qualitatively new stage in the development of accelerators. The first ideas of this kind were expressed, as research shows, by the Swedish scientist Ising in 1924, but did not lead to the creation of a workable model. The linear version of the resonant accelerator was also studied by the Swedish physicist R. A video editor who also contributed to the development of betatron. There were no fundamental flaws in their schemes, but alas, only the absence of powerful short-wave generators in the late 20s did not allow them to be implemented in practice. It has already been mentioned above about the abundance of ideas that appeared at that time, which, unfortunately, did not find technical implementation. In this regard, it is worth mentioning the name of the American engineer J. Slepyan, whose patents contain prototypes of some future accelerators, including the well-known betatron and linear resonance accelerator.
Resonant acceleration was put on a real basis in the works of E. Lawrence, conducted in the laboratory of the University of California at Berkeley. Almost simultaneously, in 1930-1932, working models of a cyclotron appeared in this laboratory – the first cyclic accelerator, in the creation of which M. Livingston played an important role, and a linear resonant accelerator with drift tubes (D. Sloan). However, linear systems soon faded into the background due to the insufficient development of microwave technology compared to the cyclotron, which has already begun its truly great triumphal march.
Already in 1935, the energy of alpha particles equal to 11 MeV was obtained and for the first time exceeded the maximum energy of natural radioactive isotopes, and in 1938 a cyclotron with a pole diameter of 1.52 m was launched, on which alpha particles with an energy of 32 MeV were obtained. Before the outbreak of World War II, the construction of a cyclotron for deuterons with an energy of 100 MeV was started. The first cyclotron in Europe was launched in Leningrad in 1936 at the Radium Institute at an energy of 6 MeV.
Speaking about the general role of the cyclotron in the development of nuclear physics, it is difficult to overestimate it. A particularly important stage was the acceleration of deuterons in the cyclotron, first because of the interest that the deuteron represents as the simplest nuclear system, and then because of the opportunities that opened up for generating intense neutron fluxes using easy-going (d-n) type reactions, that is, deuteron-neutron reactions. The significance of the last mentioned circumstance does not require comments, since thanks to it, accurate quantitative information about the cross sections of the capture and fission reactions was subsequently obtained, because reactions with neutrons attracted great attention in the future due to uranium technology.
The problem of electron acceleration stood somewhat apart and could not be solved in the way of the development of the cyclotron, which is fundamentally unsuitable for the acceleration of relativistic particles. Linear accelerators experienced their real rebirth only after the Second World War due to the rapid development of microwave oscillation generation technology for radar purposes. However, in 1940, D. Kerst launched a cyclic induction accelerator in the USA, that is, a non—resonant 2.3 MeV betatron accelerator, the main idea of which was contained in Slepyan's patents. Videoroe came close to creating a betatron, who for the first time formulated the so-called betatron condition, which makes it possible to keep the radius of the orbit almost constant during acceleration, which turned out to be important from a practical point of view. In addition, in the early 40s, the conditions for the stability of electron motion in the betatron were clearly clarified, which was of fundamental importance. The fact is that the accelerating electric field in a betatron in practical conditions turns out to be very small and in order to achieve the same energy, a particle, instead of hundreds of meters, as in a cyclotron, must travel a full path of thousands of kilometers, which, of course, is strongly affected by even small perturbations of motion.
Kerst's work was repeated, although not immediately, in several laboratories, including in the USSR, and betatron soon became a reliable and simple source of bremsstrahlung used in photonuclear reaction physics and engineering. However, the main drawback of the cyclotron is a small accelerating field, which almost inevitably follows from the non—resonant nature of acceleration, and it determined the maximum energy at 100 MeV, when the largest betatron of the University of Illinois in the USA gave an energy of 300 MeV. The fundamental nature of this limitation is related to the magnetotormotic or, more precisely, synchrotron radiation of particles moving in a circle in the vacuum chamber itself.
The theory of synchrotron radiation, developed in the early 40s and well confirmed experimentally, indicated an inevitable increase in radiation losses with energy, which could not be compensated for by the relatively small accelerating field of the betatron. Thus, in the early 40s, an outwardly dead-end situation developed: it seemed that resonant methods had reached their ceiling associated with relativistic effects, and non-resonant ones faced insurmountable technical difficulties. At the same time, the transition to the energy range of the order of hundreds of MeV was necessary due to the emergence of a new branch of science – elementary particle physics and the requirements for the generation of recently discovered mesons, when the rest energy of the μ-meson is 106 MeV, and the π-meson is as much as 140 MeV. A new qualitative stage in the history of accelerators is associated with the name of V. I. Veksler, who then worked at the Lebedev FIAN.
Used literature
1. Aliyev I. H., Sharofutdinov F. M. The use of accelerators and the phenomena of collisions of elementary particles with high-order energy to generate electrical energy. The Electron project. Monograph. Publishing solutions. Ridero. 2021. – 594 p.
2. Aliyev I. H. Software modeling of nuclear reaction phenomena based on the technology of creating a set of data using a system of algorithms in C++. The Core-COMPUTER project. Monograph. Publishing solutions. Ridero. 2022. – 156 p.
3. Aliev I. H. New parameters for nuclear reactions to be carried out on an accelerator of charged particles of the LCC-EPD-300 type. The Electron project. Monograph. Publishing solutions. Ridero. 2022. – 498 p
. 4. Aliyev I. H., Karimov Sh. B., Karimov B. H., Yuldoshaliev D. K. Development of aerator technology based on alternative energy sources Aerator project. Monograph. Publishing solutions. Ridero. 2022. – 141 p.
5. Aliyev I. H., Burnashev M. A. Ingential mathematics. Publishing solutions. Ridero. 2022. – 149 p.
6. Karimov B. H., Mirzamakhmudov T. M. Electronics asoslari. Study guide. Publishing solutions. Ridero. 2022. – 184 p.
7. I. B. Issinsky. Introduction to the physics of charged particle accelerators. A course of lectures. Edited by Ph.D. A. B. Kuznetsov. UNC-2012-52. Dubna. 2012.
8. M. Vasiliev, K. Stanyukovich. Into the depths of the inexhaustible. Atomizdat. 1975.
9. P. T. Astashenkov. The feat of Academician Kurchatov. Knowledge. Moscow. 1979.
10. A. A. Borovoy. How particles are registered. The science. 1981.
11. V. N. Dubrovsky, Ya. A. Smorodinsky, E. L. Surkov. The relativistic world. The science. 1984.
12. M. E. Levinstein, G. S. Simin. Barriers. The science. 1987.
13. L. A. Ashkinazi. A vacuum for science. The science. 1987.
TECHNICAL SCIENCES
INTRODUCTION TO THE METHOD OF PREPARATION» SUBJECT DEVELOPMENT" OF AN INNOVATIVE NATURE
UDC 377
Otajonov Salim Madrakhimovich
Doctor of Physical Sciences, head and professor of the Department "technological education" of the physics and Technical Faculty of Fergana State University
Fergana State University, Fergana, Uzbekistan
Annotatsiya. Umumiy o’rta ta’lim maktablarida texnologiya darslari maktabda eng o’zoq vaqt o’tiladigan o’quv fanlaridan biri hisoblanadi. Texnologiya o’qituvchi rahbarligida o’quvchilar tomonidan bajariladigan aqliy va jismoniy harakatlar – mehnat faoliyati jarayonidan iborat bo’lib, yakuniy natijada ularning mehnat qurollari, vositalari va jarayonlari haqida bilimlarini hamda ma’lum sohadagi ishlab chiqarish mehnatini bajarish uchun zarur amaliy ko’nikma va malakalarini egallashlariga, ongli ravishda kasb tanlashga hamda jamiyat va shaxs farovonligi yo’lida mehnat faoliyatiga qo’shilishlariga imkon beruvchi shaxsiy sifatlarini va tafakkurlarini rivojlantirishga qaratilgan o’quv fanidir.
Kalit so’zlar: innovatsiya, texnologiya, yangi metodlar, xarakteristika.
Аннотация. В общеобразовательных школах уроки технологии являются одним из самых важных предметов обучения в школе. Технология состоит из умственных и физических действий, выполняемых учащимися под руководством учителя – процесса трудовой деятельности, который в конечном итоге приводит к приобретению ими знаний об орудиях, средствах и процессах труда, а также практических навыков и умений, необходимых для выполнения производственного труда в определенной области, приобретению личностных качеств, позволяющих им осознанно выбирать профессию и включаться в трудовую деятельность на благо общества и личности. и является учебной дисциплиной, направленной на развитие мышления.
Ключевые слова: инновация, технология, новые методы, характеристика.
Annotation. In general secondary schools, technology classes are one of the academic disciplines in which the most time is spent at school. Technology consists in the process of mental and physical actions – labor activity performed by students under the guidance of a teacher, the final result of which is an educational discipline aimed at developing their personal qualities and thinking, which allows them to acquire knowledge about the tools, tools and processes of labor, as well as the necessary practical skills and abilities to perform
Keywords: innovation, technology, new techniques, characteristics.
This science is of particular importance in the activities of students and in school life, as it is taught in schools of general secondary education for a short period, that is, within the period from the first to the last grade. In this case, the lessons of technology science are organized in three stages, the purpose of which is to develop students correctly from the physical side, introduce them to the world and people of Labor, weapons and deeds of Labor, the main production areas and professions, to create labor skills related to the use of work tools, making simple items, to consciously There are specific tasks that are set before each of the above stages.
In particular, in the technology lessons of grades 1-4 at Stage 1, students are given the role of labor in the life of people, the simplest labor practices and tools of work, the first skills related to their use. By making, making simple objects, toys with the help of Labor tools such as needles, scissors, knives from materials such as paper, glue, gauze, thread, plasticine, initial labor skills are formed in them.