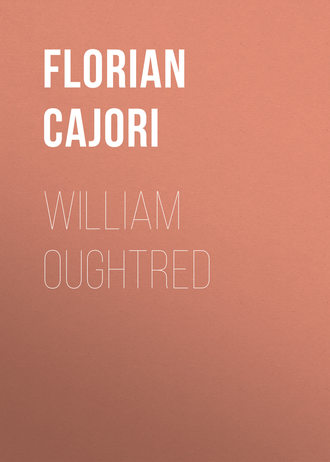
Полная версия
William Oughtred
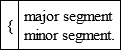
And so an equation having been proposed in which three species (terms) are in equally ascending powers, the highest species, moreover, being negative, the given magnitude which constitutes the middle species is the line to be bisected. And the given absolute magnitude to which it is equal is the rectangle beneath the unequal segments, without gnomon. As ZA-A²=AE, or in numbers, 10x-x²=21. And A or x is one of the two unequal segments. It may be found thus:
The half of the middle species is

(5), its square is

(25). From it subtract the absolute term AE (21), and

(4) will be the square of half the difference of the segments. The square root of this,
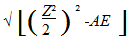
(2), is half the difference. If you add it to half the coefficient

(5), or half the line to be bisected, the longer segment is obtained; if you subtract it, the smaller segment is obtained. I say:

The quadratic equation Aq+ZA=AE receives similar treatment. This and the preceding equation, ZA-Aq=AE, constitute together a solution of the general quadratic equation, x²+ax=b, provided that E or Z are not restricted to positive values, but admit of being either positive or negative, a case not adequately treated by Oughtred. Imaginary numbers and imaginary roots receive no consideration whatever.
A notation suggested by Vieta and favored by Girard made vowels stand for unknowns and consonants for knowns. This conventionality was adopted by Oughtred in parts of his algebra, but not throughout. Near the beginning he used Q to designate the unknown, though usually this letter stood with him for the “square” of the expression after it.34
It is of some interest that Oughtred used

to signify the ratio of the circumference to the diameter of a circle. Very probably this notation is the forerunner of the π=3.14159.. used in 1706 by William Jones. Oughtred first used

in the 1647 edition of the Clavis mathematicae. In the 1652 edition he says, “Si in circulo sit 7.22::δ·π::113.355: erit δ·π::2 R.P: periph.” This notation was adopted by Isaac Barrow, who used it extensively. David Gregory35 used

in 1697, and De Moivre36 used

about 1697, to designate the ratio of the circumference to the radius.
We quote the description of the Clavis that was given by Oughtred’s greatest pupil, John Wallis. It contains additional information of interest to us. Wallis devotes chap. xv of his Treatise of Algebra, London, 1685, pp. 67-69, to Mr. Oughtred and his Clavis, saying:
Mr. William Oughtred (our Country-man) in his Clavis Mathematicae, (or Key of Mathematicks,) first published in the Year 1631, follows Vieta (as he did Diophantus) in the use of the Cossick Denominations; omitting (as he had done) the names of Sursolids, and contenting himself with those of Square and Cube, and the Compounds of these.
But he doth abridge Vieta’s Characters or Species, using only the letters q, c, &c. which in Vieta are expressed (at length) by Quadrate, Cube, &c. For though when Vieta first introduced this way of Specious Arithmetick, it was more necessary (the thing being new,) to express it in words at length: Yet when the thing was once received in practise, Mr. Oughtred (who affected brevity, and to deliver what he taught as briefly as might be, and reduce all to a short view,) contented himself with single Letters instead of those words.
Thus what Vieta would have written
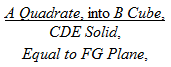
would with him be thus expressed

And the better to distinguish upon the first view, what quantities were Known, and what Unknown, he doth (usually) denote the Known to Consonants, and the Unknown by Vowels; as Vieta (for the same reason) had done before him.
He doth also (to very great advantage) make use of several Ligatures, or Compendious Notes, to signify Summs, Differences, and Rectangles of several Quantities. As for instance, Of two Quantities A (the Greater), and E (the Lesser), the Sum he calls Z, the Difference X, the Rectangle AE…
Which being of (almost) a constant signification with him throughout, do save a great circumlocution of words, (each Letter serving instead of a Definition;) and are also made use of (with very great advantage) to discover the true nature of divers intricate Operations, arising from the various compositions of such Parts, Sums, Differences, and Rectangles; (of which there is great plenty in his Clavis, Cap. 11, 16, 18, 19. and elsewhere,) which without such Ligatures, or Compendious Notes, would not be easily discovered or apprehended…
I know there are who find fault with his Clavis, as too obscure, because so short, but without cause; for his words be always full, but not Redundant, and need only a little attention in the Reader to weigh the force of every word, and the Syntax of it;.. And this, when once apprehended, is much more easily retained, than if it were expressed with the prolixity of some other Writers; where a Reader must first be at the pains to weed out a great deal of superfluous Language, that he may have a short prospect of what is material; which is here contracted for him in a short Synopsis…
Mr. Oughtred in his Clavis, contents himself (for the most part) with the solution of Quadratick Equations, without proceeding (or very sparingly) to Cubick Equations, and those of Higher Powers; having designed that Work for an Introduction into Algebra so far, leaving the Discussion of Superior Equations for another work… He contents himself likewise in Resolving Equations, to take notice of the Affirmative or Positive Roots; omitting the Negative or Ablative Roots, and such as are called Imaginary or Impossible Roots. And of those which, he calls Ambiguous Equations, (as having more Affirmative Roots than one,) he doth not (that I remember) any where take notice of more than Two Affirmative Roots: (Because in Quadratick Equations, which are those he handleth, there are indeed no more.) Whereas yet in Cubick Equations, there may be Three, and in those of Higher Powers, yet more. Which Vieta was well aware of, and mentioneth in some of his Writings; and of which Mr. Oughtred could not be ignorant.
“CIRCLES OF PROPORTION” AND “TRIGONOMETRIE”
Oughtred wrote and had published three important mathematical books, the Clavis, the Circles of Proportion,37 and a Trigonometrie.38 This last appeared in the year 1657 at London, in both Latin and English.
It is claimed that the trigonometry was “neither finished nor published by himself, but collected out of his scattered papers; and though he connived at the printing it, yet imperfectly done, as appears by his MSS.; and one of the printed Books, corrected by his own Hand.”39 Doubtless more accurate on this point is a letter of Richard Stokes who saw the book through the press:
I have procured your Trigonometry to be written over in a fair hand, which when finished I will send to you, to know if it be according to your mind; for I intend (since you were pleased to give your assent) to endeavour to print it with Mr. Briggs his Tables, and so soon as I can get the Prutenic Tables I will turn those of the sun and moon, and send them to you.40
In the preface to the Latin edition Stokes writes:
Since this trigonometry was written for private use without the intention of having it published, it pleased the Reverend Author, before allowing it to go to press, to expunge some things, to change other things and even to make some additions and insert more lucid methods of exposition.
This much is certain, the Trigonometry bears the impress characteristic of Oughtred. Like all his mathematical writings, the book was very condensed. Aside from the tables, the text covered only 36 pages. Plane and spherical triangles were taken up together. The treatise is known in the history of trigonometry as among the very earliest works to adopt a condensed symbolism so that equations involving trigonometric functions could be easily taken in by the eye. In the work of 1657, contractions are given as follows: s=sine, t=tangent, se=secant, s co=cosine (sine complement), t co=cotangent, se co=cosecant, log=logarithm, Z cru=sum of the sides of a rectangle or right angle, X cru=difference of these sides. It has been generally overlooked by historians that Oughtred used the abbreviations of trigonometric functions, named above, a quarter of a century earlier, in his Circles of Proportion, 1632, 1633. Moreover, he used sometimes also the abbreviations which are current at the present time, namely sin=sine, tan=tangent, sec=secant. We know that the Circles of Proportion existed in manuscript many years before they were published. The symbol sv for sinus versus occurs in the Clavis of 1631. The great importance of well-chosen symbols needs no emphasis to readers of the present day. With reference to Oughtred’s trigonometric symbols. Augustus De Morgan said:
This is so very important a step, simple as it is, that Euler is justly held to have greatly advanced trigonometry by its introduction. Nobody that we know of has noticed that Oughtred was master of the improvement, and willing to have taught it, if people would have learnt.41
We find, however, that even Oughtred cannot be given the whole credit in this matter. By or before 1631 several other writers used abbreviations of the trigonometric functions. As early as 1624 the contractions sin for sine and tan for tangent appear on the drawing representing Gunter’s scale, but Gunter did not use them in his books, except in the drawing of his scale.42 A closer competitor for the honor of first using these trigonometric abbreviations is Richard Norwood in his Trigonometrie, London, 1631, where s stands for sine, t for tangent, sc for sine complement (cosine), tc for tangent complement (cotangent), and sec for secant. Norwood was a teacher of mathematics in London and a well-known writer of books on navigation. Aside from the abbreviations just cited Norwood did not use nearly as much symbolism in his mathematics as did Oughtred.
Mention should be made of trigonometric symbols used even earlier than any of the preceding, in “An Appendix to the Logarithmes, shewing the practise of the Calculation of Triangles, etc.,” printed in Edward Wright’s edition of Napier’s A Description of the Admirable Table of Logarithmes, London, 1618. We referred to this “Appendix” in tracing the origin of the sign ×. It contains, on p. 4, the following passage: “For the Logarithme of an arch or an angle I set before (s), for the antilogarithme or compliment thereof (s*) and for the Differential (t).” In further explanation of this rather unsatisfactory passage, the author (Oughtred?) says, “As for example: sB+BC=CA. that is, the Logarithme of an angle B. at the Base of a plane right-angled triangle, increased by the addition of the Logarithm of BC, the hypothenuse thereof, is equall to the Logarithme of CA the cathetus.”
Here “logarithme of an angle B” evidently means “log sin B,” just as with Napier, “Logarithms of the arcs” signifies really “Logarithms of the sines of the angles.” In Napier’s table, the numbers in the column marked “Differentiae” signify log sine minus log cosine of an angle; that is, the logarithms of the tangents. This explains the contraction (t) in the “Appendix.” The conclusion of all this is that as early as 1618 the signs s, s*, t were used for sine, cosine, and tangent, respectively.
John Speidell, in his Breefe Treatise of Sphaericall Triangles, London, 1627, uses Si. for sine, T. and Tan for tangent, Se. for secant, Si. Co. for cosine, Se. Co. for cosecant, T. Co. for cotangent.
The innovation of designating the sides and angles of a triangle by A, B, C, and a, b, c, so that A was opposite a, B opposite b, and C opposite c, is attributed to Leonard Euler (1753), but was first used by Richard Rawlinson of Queen’s College, Oxford, sometimes after 1655 and before 1668. Oughtred did not use Rawlinson’s notation.43
In trigonometry English writers of the first half of the seventeenth century used contractions more freely than their continental contemporaries; even more freely, indeed, than English writers of a later period. Von Braunmühl, the great historian of trigonometry, gives Oughtred much praise for his trigonometry, and points out that half a century later the army of writers on trigonometry had hardly yet reached the standard set by Oughtred’s analysis.44 Oughtred must be credited also with the first complete proof that was given to the first two of “Napier’s analogies.” His trigonometry contains seven-place tables of sines, tangents, and secants, and six-place tables of logarithmic sines and tangents; also seven-place logarithmic tables of numbers. At the time of Oughtred there was some agitation in favor of a wider introduction of decimal systems. This movement is reflected in those tables which contain the centesimal division of the degree, a practice which is urged for general adoption in our own day, particularly by the French.
SOLUTION OF NUMERICAL EQUATIONS
In the solution of numerical equations Oughtred does not mention the sources from which he drew, but the method is substantially that of the great French algebraist Vieta, as explained in a publication which appeared in 1600 in Paris under the title, De numerosa potestatum purarum atque adfectarum ad exegesin resolutione tractatus. In view of the fact that Vieta’s process has been described inaccurately by leading modern historians including H. Hankel45 and M. Cantor,46 it may be worth while to go into some detail.47 By them it is made to appear as identical with the procedure given later by Newton. The two are not the same. The difference lies in the divisor used. What is now called “Newton’s method” is Newton’s method as modified by Joseph Raphson.48 The Newton-Raphson method of approximation to the roots of an equation f(x)=0 is usually given the form a-[f(a)/f´(a)], where a is an approximate value of the required root. It will be seen that the divisor is f´(a). Vieta’s divisor is different; it is
|f(a+s₁) -f(a)|-s₁ⁿ,where f(x) is the left of the equation f(x)=k, n is the degree of equation, and s₁ is a unit of the denomination of the digit next to be found. Thus in x³+420000x=247651713, it can be shown that 417 is approximately a root; suppose that a has been taken to be 400, then s₁=10; but if, at the next step of approximation, a is taken to be 410, then s₁=1. In this example, taking a=400, Vieta’s divisor would have been 9120000; Newton’s divisor would have been 900000.
A comparison of Vieta’s method with the Newton-Raphson method reveals the fact that Vieta’s divisor is more reliable, but labors under the very great disadvantage of requiring a much larger amount of computation. The latter divisor is accurate enough and easier to compute. Altogether the Newton-Raphson process marks a decided advance over that of Vieta.
As already stated, it is the method of Vieta that Oughtred explains. The Englishman’s exposition is an improvement on that of Vieta, printed forty years earlier. Nevertheless, Oughtred’s explanation is far from easy to follow. The theory of equations was at that time still in its primitive stage of development. Algebraic notation was not sufficiently developed to enable the argument to be condensed into a form easily surveyed. So complicated does Vieta’s process of approximation appear that M. Cantor failed to recognize that Vieta possessed a uniform mode of procedure. But when one has in mind the general expression for Vieta’s divisor which we gave above, one will recognize that there was marked uniformity in Vieta’s approximations.
Oughtred allows himself twenty-eight sections in which to explain the process and at the close cannot forbear remarking that 28 is a “perfect” number (being equal to the sum of its divisors, 1, 2, 4, 7, 14).
The early part of his exposition shows how an equation may be transformed so as to make its roots 10, 100, 1000, or 10m times smaller. This simplifies the task of “locating a root”; that is, of finding between what integers the root lies.
Taking one of Oughtred’s equations, x⁴-72x³+238600x=8725815, upon dividing 72x³ by 10, 238600x by 1000, and 8725815 by 10,000, we obtain x⁴-7·2x³+238·6x=872·5. Dividing both sides by x, we obtain x³+238·6-7·2x²=x)872·5. Letting x=4, we have 64+238·6-115·2=187·4.
But 4)872·5(218·1; 4 is too small. Next let x=5, we have 125+238·6-180=183·6.
But 5)872·5(174·5; 5 is too large. We take the lesser value, x=4, or in the original equation, x=40. This method may be used to find the second digit in the root. Oughtred divides both sides of the equation by x², and obtains x²+x)238600-72x=x²)8725815. He tries x=47 and x=48, and finds that x=47.
He explains also how the last computation may be done by logarithms. Thereby he established for himself the record of being the first to use logarithms in the solution of affected equations.
As an illustration of Oughtred’s method of approximation after the root sought has been located, we have chosen for brevity a cubic in preference to a quartic. We selected the equation x³+420000x=247651713. By the process explained above a root is found to lie between x=400 and x=500. From this point on, the approximation as given by Oughtred is as shown on p. 43.
In further explanation of this process, observe that the given equation is of the form Lc+CqL=Dc, where Lc is our x, Cq=420000, Dc=247651713. In the first step of approximation, let L=A+E, where A=400 and E is, as yet, undetermined. We have
Lc=(A+E)³=A³+3A²E+3AE²+E³
and
CqL=420000(A+E).
Subtract from 247651713 the sum of the known terms A³ (his Ac) and 420000 A (his CqA). This sum is 232000000 the remainder is 15651713.
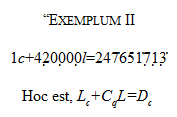
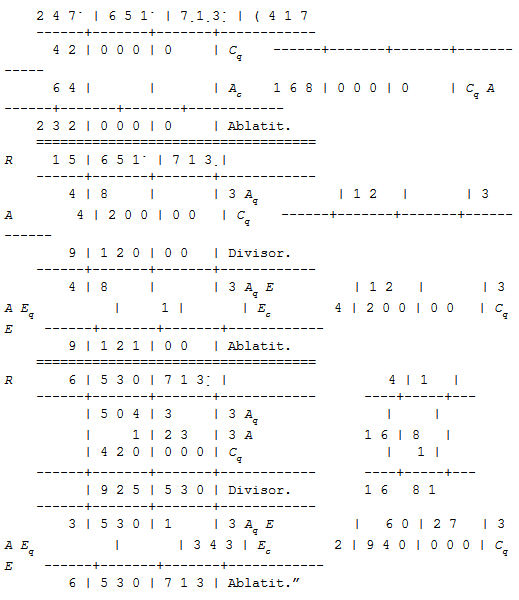
Next, he evaluates the coefficients of E in 3A²E and 420000E, also 3A, the coefficient of E². He obtains 3A²=480000, 3A=1200, Cq=420000. He interprets 3A² and Cq as tens, 3A as hundreds. Accordingly, he obtains as their sum 9120000, which is the divisor for finding the second digit in the approximation. Observe that this divisor is the value of |f(a+s₁) -f(a)|-s₁ⁿ in our general expression, where a=400, s₁=10, n=3, f(x)=x³+420000x.
Dividing the remainder 15651713 by 9120000, he obtains the integer 1 in ten’s place; thus E=10, approximately. He now computes the terms 3A²E, 3AE² and E³ to be, respectively, 4800000, 120000, 1000. Their sum is 9121000. Subtracting it from the previous remainder, 15651713, leaves the new remainder, 6530713.
From here on each step is a repetition of the preceding step. The new A is 410, the new E is to be determined. We have now in closer approximation, L=A+E. This time we do not subtract A³ and CqA, because this subtraction is already affected by the preceding work.
We find the second trial divisor by computing the sum of 3A², 3A and Cq; that is, the sum of 504300, 1230, 420000, which is 925530. Again, this divisor can be computed by our general expression for divisors, by taking a=410, s₁=1, n=3.
Dividing 6530713 by 925530 yields the integer 7. Thus E=7. Computing 3A²E, 3AE², E³ and subtracting their sum, the remainder is 0. Hence 417 is an exact root of the given equation.
Since the extraction of a cube root is merely the solution of a pure cubic equation, x³=n, the process given above may be utilized in finding cube roots. This is precisely what Oughtred does in chap. xiv of his Clavis. If the foregoing computation is modified by putting Cq=0, the process will yield the approximate cube root of 247651713.
Oughtred solves 16 examples by the process of approximation here explained. Of these, 9 are cubics, 5 are quartics, and 2 are quintics. In all cases he finds only one or two real roots. Of the roots sought, five are irrational, the remaining are rational and are computed to their exact values. Three of the computed roots have 2 figures each, 9 roots have 3 figures each, 4 roots have 4 figures each. While no attempt is made to secure all the roots – methods of computing complex roots were invented much later – he computes roots of equations which involve large coefficients and some of them are of a degree as high as the fifth. In view of the fact that many editions of the Clavis were issued, one impression as late as 1702, it contributed probably more than any other book to the popularization of Vieta’s method in England.