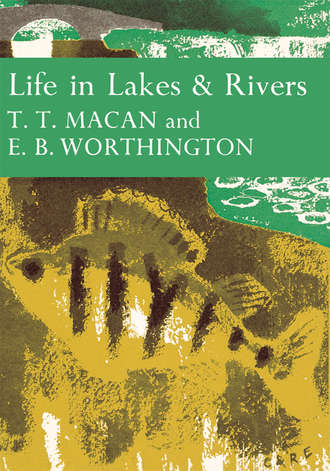
Полная версия
Life in Lakes and Rivers
On the whole there is a serial increase or decrease in each of the three columns. The most notable anomaly is the low transparency of Bassenthwaite, occasioned by its being the only lake of which the water is stained with peat. The amount of light at different depths in Bassenthwaite, Windermere and Ennerdale is shown in Figure 11, expressed as a percentage of the intensity at the surface.
Table 2. The sequence of Lake District Lakes (Pearsall, 1921)
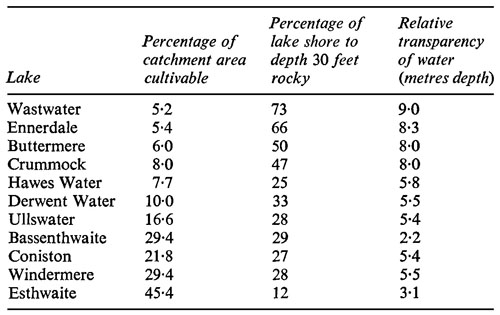
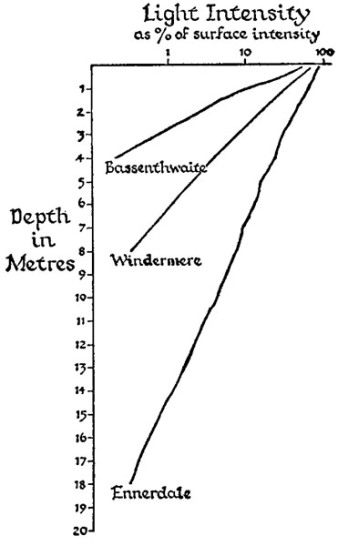
Fig. 11 Penetration of light into three Lake District lakes
Work on cores, started just before the war, had as its original aim the elucidation of the history of the lakes, but, like many another new line in research, an essentially opportunist activity, it proved most fruitful in a line other than the one aimed at and it revealed more about the land than about the water. However, as a lake is strongly influenced by events in the drainage area, the findings are relevant. Most animals disappear completely, but the shells of some waterfleas (Cladocera) and the heads of some chironomids do not decompose and persist in the cores. Similarly many algae leave no trace, but the siliceous skeletons of diatoms (e.g. Asterionella) that have lain in the mud for thousands of years are still identifiable. In contrast the pollen of nearly every plant that produces any does not decompose and, since that of almost all species is distinct, an examination of cores gives a picture of what the land flora was like when the particular layer of mud under examination was deposited. Research on pollen in cores from bogs and other places where soil has been accumulating since the Ice Age was in vogue all over Europe at the time, which was fortunate, because events in the lake cores could be related to events elsewhere, and some of these had been dated by one means or another. Chemical analysis of cores also yielded a large amount of information about the past.
The lower part of a core from Windermere consists of clay which, on examination, proves to be made up of alternating layers of very fine and coarser particles; it is accordingly referred to as laminated clay by Dr Winifred Pennington, who has described the cores. Above the laminated clay, which is pink, lies a grey layer and above that more pink clay, which may or may not be laminated. On top of this is a thick column of brown mud which extends nearly to the surface; it is capped by a fourth kind of soil, a black deposit which Pennington refers to as ooze.
The laminated clay contains very little organic matter and few remains of plants, and was almost certainly formed towards the end of a glacial period, for similar deposits are being laid down today in certain glacier-fed lakes. During the summer both coarse and very fine particles are washed into the lake. The coarse particles sink almost immediately but the fine particles remain in suspension for a long time. When winter comes the inflowing streams freeze, and so no particulate matter is brought into the lake, but fine particles left over from the summer are still settling. The result is a summer layer of coarse and fine particles and a winter layer of fine particles only.
The low organic content of this deposit and the scarcity of plant remains indicate that there was little life in the lake when the laminated clay was being laid down. In contrast the grey mud contains the remains of animals and plants, and the lake was evidently more densely populated during the period when it was being laid down. These organisms were associated with an improvement in the climate, which is known as the Allerød period, because it was first discovered near the place of that name. It was followed by a return of glacial conditions when the pink clay with few remains of organisms was laid down again. Professor H. Godwin has had dated by means of the C14 method, a technique which will no doubt be more widely used when facilities for it are more widely and more easily available, a sample from the Allerød layer and found it to be some 12,000 years old. The ooze at the top in which Asterion-ella suddenly becomes common obviously represents enrichment of some kind. Today the effluents from the sewage works are probably the main sources of enrichment, but this is recent. The population, particularly the holiday-making one, has been increasing since the railway came to Windermere in 1847, but the transition from earth closets to running water sanitation has been slower, and it is doubtful if the lake was being seriously enriched from this source a century ago. If mud is being deposited on the bottom of Windermere at a rate that has been constant over the last few hundred years, whatever caused Asterionella to appear happened about two centuries ago. It therefore probably antedates the tourist completely, and is to be sought in improved agricultural practice of which there is some evidence early in the eighteenth century.
Mackereth’s conclusions from chemical analyses upset certain ideas of long standing. The lakes were richest chemically in their earliest days, when the land was covered with rock fragmented by the ice and exposing a great area of surface from which the rain could leach nutrient salts. Esthwaite was eutrophic at an early stage and presumably, therefore, the lakes were richest biologically when they were richest chemically. Some of the algal species identified in the cores support this view. The rocks are hard and when a fresh surface has been leached for a time water dissolves little from it. The lakes slowly became less productive. A climatic change and the arrival of man, who started to fell trees, resulted in more erosion and enriched the lakes, but as more stable conditions were established production fell again. A thousand years ago the Norsemen arrived, and since then man has been the most important agent affecting the lakes. The increased production in some lakes on account of their situation has already been described.
CHAPTER 5
RIVERS

It is possible to entitle a chapter ‘a typical lake’ and to fill it with an account of physical and chemical changes which follow an annual cycle in a great many lakes. Another chapter is entitled ‘different kinds of lakes’, though here a certain degree of accuracy has been sacrificed to obtain a short title, and the account includes pieces of water that are too small to be regarded as lakes in the strict sense. In neither chapter is there much about fauna and flora, the plan being to describe freshwater animals and plants in chapter 6 and then to pass to an account of the various communities found in different biotopes. A biotope is a region in which the conditions are of such uniformity that the plant and animal communities do not vary much; the stony substratum of a lake, weed-beds and the open water are examples of biotopes. The purist would prefer the term biocoenosis for the assemblage of animals and plants that inhabit a biotope, but here the more general term ‘community’ is used.
It is impossible to treat rivers in the same way. Lakes are all recent in geological terms, and many of what were called lakes in the preceding chapter are recent in historical terms, having been made by man. Each lake was formed by one event taking place in a limited area. Water courses are much older and have continued their existence in spite of the events which formed lakes. For example the rivers of the English Lake District tend to rise near the middle of the area and radiate from it. This pattern was presumably established at a time when the mountains exposed today were covered by a dome of younger rocks. The watercourses cut down through this and later eroded the underlying rocks in the same direction, though these originally had the form of a ridge not a dome. The disappearance of the covering layers left the old rocks scarred by valleys whose direction bears no relation to the way in which they were laid down. Each watercourse is, therefore, modified today by many geological strata.
The plan of this chapter is to describe the few rivers about which something is known; how far they are ‘typical’ only further work will show. It has also been found necessary to include some information about the plants, and about human activities. Whereas these have brought whole new bodies of standing water into existence, they have modified rivers. These modifications, however, have been extensive and have affected every British river of large size. Waste disposal, water supply, water power, drainage and navigation have been the main activities through which man has altered water-courses.
The history of investigation of rivers fits more easily into the account of their misuse in Chapter 14. It suffices to record here that important surveys were carried out between the wars by the late Mr F. T. K. Pentelow, Dr R. W. Butcher and colleagues in the Ministry of Agriculture team. Since the war knowledge about small stony streams has advanced greatly, investigations having been made in most of the upland areas of England, Wales and Scotland. One Lake District river has been investigated by Drs R. Kuehne and W. Minshall working in England during the tenure of a year’s fellowship from America. Of recent years the number of biologists on the staffs of the River Authorities has increased considerably. It seems to be envisaged, however, that their task is to analyse as many samples as possible from as many stations as possible in order to keep a check on the condition of the river and its tributaries. For this purpose it is often reckoned that identification to species is not necessary. For basic information about the lower courses of rivers and their inhabitants, we still have to turn to the old surveys.
The property of water important to the study of lakes is its density at different temperatures; for the study of rivers, its flow downhill. This has a direct influence on organisms that live in it and a possibly more important indirect one through its effect on the substratum. Table 3 is taken from Tansley (1939) but its original source is a text-book on river and canal engineering. An engineer contrives even gradients and neatly regulated bends; Nature does not. The irregularity of a natural water-course produces a mozaic that confounds the systematic mind at the start and ensures that any scheme of classification is no more than a rough general guide. What does happen in nature? In attempting to answer that question, we shall take an imaginary river, but admit at once that our imaginations owe much to familiarity with the Lake District. The rocks there are hard, impermeable and often steep. In places water flows a long distance over a flat sheet of rock, but generally it has eroded a gulley of some kind. In this rapids generally alternate with pools in parts of which conditions are surprisingly quiet, especially if the stones and boulders are large. In the rapids large stones and boulders tend to jam in the gulley and hold up a bottom that is far more stable than might be expected on so steep a gradient. Conditions in this zone must obviously depend on the relation between the dip of the strata and the angle at which they are exposed, and on the size and form of the fragments which break off the rock. Where the gradient becomes less steep, moderate-sized stones plucked from the rocks above and washed down begin to come to rest. In the Lake District they tend to be flat and accordingly they have an inherent stability. Further breaking-up is taking place all the time and as the smaller pieces are rolled downstream their edges are rounded. This produces the unstable bottom of round stones that is often found some distance down the valley, if the gradient falls evenly. An outcrop of rock is a fresh source of flat stones, and it, or any other obstruction, produces a striking alteration of the flow pattern, confining swift current to the surface layers. Settling of gravel, sand and finer particles becomes possible, and, as these fill up the interstices between the larger stones, they produce a remarkably stable bottom. This happens also during a spell of low water. Percival and Whitehead refer to it as a ‘cemented’ bottom. It is one which occurs almost everywhere, but generally it is covered by loose stones. Occasionally some new obstruction halts the downward flow of loose stones and then the stream is floored by substratum of this type.
Table 3. Relation of current speed and nature of river bed
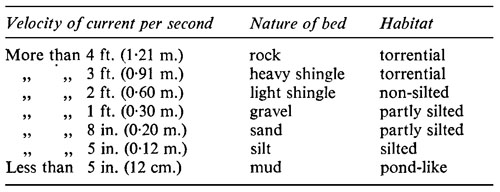
Flowing onward, the river generally comes to a plain, often one of its own creating, the gradient approaches nearer and nearer to the horizontal and flow decreases. First gravel, then sand and finally silt settle to the bottom and provide a substratum in which plants can take root.
Probably few except those charged with the task of dredging it realize how much material is being deposited on river beds. It is not a continuous process and varies greatly with intensity of rainfall. At intervals exceptional downpours, often restricted to a comparatively small area of the mountains, make considerable alterations to a river bed, which may remain comparatively unchanged until the next downpour. But change never wholly stops; a boulder may stabilize a stretch for many years but all the time it is being chipped away by the smaller stones washed past it until the day must come when it is no longer large enough to withstand a flood. Away it goes and a considerable section of the adjacent bottom with it until a new pattern is established.
For the biologist the important distinction is between the upland reaches, where erosion is taking place, and only plants, such as mosses, that can attach themselves to flat hard surfaces provide cover for animals, and the lowland reaches where a plain is being built (or would be if the drainage engineers permitted) and rooted vegetation grows. Dudley Stamp (1946) recognizes three zones; mountain, foothill, and plain. Butcher has proposed a classification of rivers according to which of these zones they rise in. In mountain areas with hard rocks the rivers will traverse all three zones, but where there is chalk or other pervious rock the river may spring from the foothill or the plain region. His scheme, however, has not caught on, and most workers agree that an entire river may be so diverse that it will not fit with other rivers into a category. Schemes for recognizing zones within a river have, in contrast, been popular. The best known goes back a long way and has been elaborated in recent years especially by fishery workers. It is based on the species of fish found, which appears to have a fair correlation with the slope. One drawback is that some of the fish do not have a wide geographical distribution. Dr Kathleen Carpenter (1928) has adapted it for British waters:
1. The Headstreams and Highland Brooks are small, often torrential, and without fish. Temperature conditions vary greatly. Low temperature is common, but a stream that arises from shallow soil may be warm, and a slow-flowing stretch may soon reach a high temperature on a sunny day.
2. The Troutbeck is larger and more constant than the headstream. Torrential conditions are typical and the bottom is composed of solid rock, stones, and boulders, with perhaps some gravel. The trout is the only permanent fish of the open water though the miller’s thumb (Cottus gobio) is found sheltering among stones. These first two zones together correspond roughly with Stamp’s upper or mountain course.
3. The Minnow Reach is still fairly swift and patches of silt and mud are only to be found in a few places protected from the current, but higher plants, notably the water crowfoot, Ranunculus fluitans, are able to gain a foothold. This is roughly the middle course of Stamp. It is the Thymallus (grayling) zone of the continental workers, but Carpenter rejects this name because the grayling is not a widespread species in Britain.
4. The Lowland Reach is slow and meandering, with a muddy bottom and plenty of vegetation. Coarse fish are characteristic, and on the continent of Europe it is known as the bream zone.
Tansley classifies rivers into five zones, basing his system very largely on the work of Butcher (1933).
Zone 1 is described as very rapid. Where vegetation is present at all, the important plants are mosses and liverworts; higher plants are often absent altogether and never dominant. This class includes all Carpenter’s headstreams and highland brooks and part, at least, of the troutbeck.
Zone 2 is moderately swift with a bottom of stones and boulders, but with occasional patches of finer material in which a small number of higher plants can gain a foothold. Ranunculus fluitans (or sometimes R. pseudofluitans), the water crowfoot, is the most important.
Zone 3 has a moderate current with a gravelly bed. The list of higher plants is much longer. The water crowfoot is still the most important, others are the simple bur-reed, Sparganium simplex, several species of Potamogeton, and the Canadian pond-weed, Elodea canadensis.
Zones 4 and 5 are medium to slow, and very slow or negligible respectively. The list of higher plants is long and, as it varies a good deal from river to river, confusion rather than clarification would be the result of reproducing it here; but it may be noted the water crowfoot is usually not an important constituent. It is impossible to equate this classification exactly with that of Carpenter, but Zone 2 and part, at least, of Zone 3, correspond with her minnow reach, and Zones 4 and 5 and perhaps part of Zone 3 correspond with her lowland coarse fish reach.
Against this background a few British rivers which have been studied in detail may now be examined. The Lake District, as was described earlier, is drained by rivers which radiate from the centre. Their valleys were enlarged by glaciers during the Ice Age and generally deepened in such a way that a lake was left when the ice retreated. The Duddon is one of the few valleys in which there is no lake. Its highest tributary, Gait-scale Gill, rises at an altitude of 735 m. (2400 ft.) in a flat area covered with bog and small pools of open water. Beyond this it tumbles steeply down the fellside, dropping 300 m. in 900 m. (33%). At the foot of this slope there is a delta of large stones under which the water disappears in dry periods. Exceptional rainfall towards the end of the year during which Kuehne and Minshall were at work enlarged this delta, and incidentally carried away every one of about twelve maximum and minimum thermometers which they had buried in various parts of the system. The analyses made by these two workers showed that the calcium ranged from 0.57 to 1.10 parts per million in Gaitscale Gill, a low value, even for the Lake District, but after the flood the concentration below the delta rose to 3.0 p.p.m. This illustrates the point, stressed earlier, that freshly exposed faces yield more nutrients than those which have been leached for some time. The highest temperature recorded in the gill was 17.2° C., 5.6° C. lower than the highest temperature recorded elsewhere in the system.
Several streams run down the fellside parallel with Gaitscale Gill and feed the main river which here runs roughly westwards. Beside it runs a road, originally made by the Romans, probably as a line of communication through the area to facilitate the subjection of the natives (Rollinson, 1967). It is now used mainly by tourists, for there are no dwellings beside it between Langdale at one end and Eskdale at the other. The river swings round to take a southerly direction in an upper valley with a comparatively slight incline. There were four farms in this valley, but only two are used for farming today. In autumn a few green fields around them stand out among the predominant greyish-yellow of the poorer pastures, probably as a result of liming. Sheep, which range far and wide over the surrounding fells, particularly in summer, are the chief product. A few conifers, planted recently, are the only trees.
The slope steepens to separate the lower from the upper valley. The slope in the lower valley is 1 %, the river is floored with round stones of all sizes, and it flows torrentially down to the estuary. There is no plain region, and only the first two of Carpenter’s four zones and the first of Butcher’s five can be recognized. There are two small villages in the lower valley, residences scattered outside them, and some twelve farms on which cattle as well as sheep are reared. Deciduous woods cover extensive areas of the valley sides. The upper valley comes to an end about 175 m. above sea level, the lower at sea level. The difference in climate between the two is obviously great but no figures are available. The temperature of the swift river gives no indication of it. The valley walls rise steeply on both sides but to the west there is a plateau over which flows the longest tributary.
The River Duddon is about 11 miles (18 km.) long. We pass from it to the River Tees (Butcher, Longwell, and Pentelow, 1937) which is about 100 miles (160 km.) long. It rises in the Pennines and flows to the North Sea. The gathering ground drained by the headstreams is fairly large. It is high above sea level, it receives a relatively heavy rainfall of about 60 inches (1520 mm.) a year, and the rock is impermeable. The result of these four factors is a severe scour in time of flood, and this has carved out a deep bed. Consequently the energy of a flood is not dissipated in inundating the surrounding country and the effect is concentrated on the river-bed. On one occasion some carts were being filled with gravel at the water’s edge when the river rose so suddenly that the carts had to be abandoned and were swept away. This illustrates a most important condition affecting the plants and animals of the river.
The small town of Croft is about 45 miles (70 km.) from the mouth and about 65 miles (100 km.) from the source, travelling by river, and is a convenient dividing point. Above Croft all the river is rapid with little rooted vegetation and few fish except trout, grayling, and minnows. The river downstream is still moderately swift but there is much more rooted vegetation and various coarse fish are plentiful. After flowing for 20 miles (32 km.) from Croft the river reaches the head of the estuary, which is some 25 miles (40 km.) long.
The Tees rises on Cross Fell at a point about 2,500 feet (760 m.) above sea level. Many small tributaries also rise just on the eastern side of the Pennine watershed, and some of them originate in thick peat beds. One, for example, drains the peat pools which were mentioned in Chapter 4. These little streams run down the hillside with a fairly though not extremely rapid flow, because the eastern slopes of the Pennines are not steep. About six miles from the source three main tributaries have coalesced and the river is some 12 metres wide with a fair flow over a bottom of stones and boulders. Then it enters a quiet stretch and for three miles the current is sufficiently slow to allow the deposition of some fine sediment, which provides a foothold for a few higher plants, Potamogeton alpinus, the alpine pond-weed, Callitriche intermedia, the water starwort, and Sparganium simplex, the simple bur-reed. The only other attached plants are the mosses, Fontinalis antipyretica, and Eurhynchium rusciforme, and, at certain seasons, the algae Lemanea fluviatilis and Cladophora glomerata.
This slow stretch provides a pretty example of the sort of exception to the general plan which is to be found in almost any river. It is caused by a stratum of hard rock, and at the end of it there is a fine waterfall. A little farther on, some 16 miles (20 km.) from the source and 1,000 feet (305 m.) above sea level, the river strikes a road and some human habitations, and comes to the end of what may conveniently be taken as the first part of its course. A chemical station was set up just here and the results obtained are shown in Table 4.