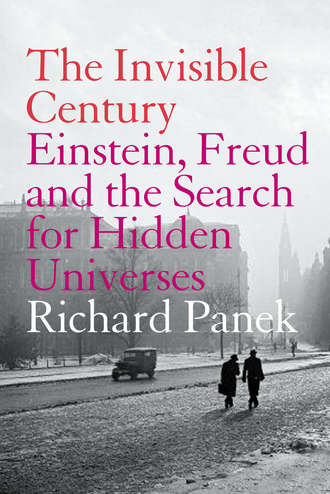
Полная версия
The Invisible Century: Einstein, Freud and the Search for Hidden Universes
He tried it. First Einstein proposed that just as Newton’s mechanics don’t allow observers either on a dock or on a ship to consider themselves to be the ones absolutely at rest, neither should electrodynamics and optics. “We shall raise this conjecture (whose content will hereafter be called ‘the principle of relativity’) to the status of a postulate,” he wrote in the second paragraph of his paper. Then he accepted the constancy of the speed of light in empty space as another given—a second postulate: that the speed of light in a vacuum is always the same “independent of the state of motion of the emitting body.”
And that was all. It worked. Einstein now had two mutually reinforcing postulates, “only apparently irreconcilable”: a principle of relativity, allowing us to conduct experiments involving light either on the ship or on the dock with equal validity; and a principle of constancy, allowing the ship (or the dock, for that matter) to approach the speed of light without any light onboard (or on the dock)—including electromagnetic waves bringing images from objects to our eyes—slowing to a stop and thereby revealing whether the ship (or dock) is the one “really” in motion. In which case, as Einstein promised his readers in that same second paragraph, the “introduction of a ‘light ether’ will prove to be superfluous, inasmuch as the view to be developed here will not require a ‘space at absolute rest’ endowed with special properties.”
The rest was math—high-school-level algebra, at that. Suppose that you’re standing on a dock watching our old Galilean ship, still anchored just offshore after all these centuries. And suppose that the ship is absolutely motionless in the water. And suppose that instead of dropping a stone, someone on board is dropping a light signal—sending a beam of light from the top of the mast to the deck. If you time this simple event and get an answer of, say, one second, then you know that the distance between the top of the mast and the deck must be the distance that light travels in one second, or 186,282 miles. (It’s a big ship.)
The complications begin, just as they did in Galileo’s day, once that ship lifts anchor and sets sail. Suppose that it’s moving at a constant speed across your line of sight. If the person at the top of the mast sends a second light signal in the same manner as the first, what will you see from your vantage on the dock? The Aristotelian answer: a streak of light heading straight for the center of the Earth and therefore landing some distance behind the base of the mast—a distance corresponding to how far the ship has traveled along the water during the signal’s journey. The Galilean answer: a streak of light heading straight for the base of the mast—which is the Einsteinian answer as well. From your point of view, the base of the mast will have moved out from under the top of the mast during the descent of the beam of light, just as it did during the descent of a stone. Which means the distance the light has traveled, from your point of view, has lengthened. It’s not 186,282 miles. It’s more.
How much more you can easily find out by measuring the time of its journey—and that’s where the Einsteinian interpretation begins to depart from the Galilean. What is velocity? Nothing but distance divided by time, whether inches divided by—or, in the vernacular, per—day or kilometers per hour or miles per second. But if we accept Einstein’s second postulate, then the velocity in question isn’t just 186,282 miles per second. It’s always 186,282 miles per second. It’s constant—indeed, a constant. In the equation “velocity equals distance divided by time,” this constant is over on one side of the equals sign, off by itself, humming along at its own imperturbable rate. On the opposite side of the equals sign are the parts of the equation that can vary, that are indeed the variables—distance and time, also known as miles and seconds. They can undergo as many permutations as you can imagine, as long as they continue to divide in such a way that the result is 186,282 miles per second, or the equivalent—372,564 miles per two seconds, or 558,846 miles per three seconds, or 1,862,820 per ten seconds, and so on. Change the distance, and you have to change the time.
You have to change the time.
For more than two centuries, though, you didn’t. Now, on an evening in May 1905, you suddenly did, because on that evening Einstein, having talked the problem through with his friend Besso, realized that he needed to take into account something he’d never before adequately considered: the “inseparable connection between time and the signal velocity.” Time was a variable, a measurement that passed at different rates according to where you were. To an observer on the dock, the second light signal would had to have lasted longer than one second. To an observer on the ship, however, the second light signal would have appeared to do what the first one had done, back when the ship was anchored in the water: travel straight down to the base of the mast, 186,282 miles away. For this observer, the distance wouldn’t have differed from one signal to the next, so the time wouldn’t have, either. The shipboard observer would be measuring one second while you on the dock would have been counting two seconds or three seconds or more, depending on the speed of the ship along the water. For this reason, you would have every right to say that clocks on board the ship were moving slowly. And there it is: a new principle of relativity.
Of course, such an effect wouldn’t become noticeable unless the ship were moving at a significant fraction of the speed of light. At more modest speeds, the Galilean interpretation holds to a high degree of accuracy; as Einstein later wrote, “it supplies us with the actual motion of the heavenly bodies with a delicacy of detail little short of wonderful.” Still, according to Einstein’s math, as long as a ship is in motion at all, the distance the light travels on its angular path would have to be greater than the simple perpendicular drop you would see when the ship is at rest relative to you, and therefore the time to cover that distance would have to be greater, too. Through similar reasoning, Einstein also established that for an observer in a relative state of rest, back on the dock, the measurement of the length of a rod aboard a moving ship would have to shorten in the direction of motion, and to grow shorter the faster the ship is moving relative to the dock. And vice versa: Someone on the supposedly moving ship would have every right to consider that system to be the one at rest, and you and your so-called resting system to be the one whose dimensions would also appear to have shortened, and whose time would also appear to have slowed.
So which observer would be “right”? The observer on the ship, or you on the dock? The answer: Both—or, maybe more accurately, either, depending on who’s doing the measuring. But how much time passed really? How long is the rod really? The answer: There is no “really”—no absolute space, no ether, against which to measure the motions of all matter in the universe. There is only the relative motions of the two systems.
“For the rest of my life I want to reflect on what light is,” Einstein once said. If Einstein were correct, the universe wasn’t quite a clockwork mechanism; it didn’t function only according to the visible motions of matter. Instead it was electromagnetic, operating according to heretofore hidden principles. On a fundamental level, it was less a pocket watch than a compass.
Not that this new understanding of the universe was complete. Einstein knew that all he’d done was take into account the measurements of objects moving at uniform, or nonvarying, velocities relative to one another—a highly specialized situation. He hadn’t yet taken into account the measurements of objects moving at nonuniform, or varying, velocities relative to one another—a far more representative sampling of the universe as we know it.
Still, it was a start. In a way, Einstein’s light-centered universe was as physically distinct from the Galilean one he’d inherited as Galileo’s sun-centered universe was from the Aristotelian one he’d inherited. But like Galileo, Einstein knew his had to be true—or truer than the one it was replacing, anyway—because he had seen the evidence for himself, if only in his mind’s eye.
TWO MORE THINGS ON EARTH
Listen.
And so the boy listened. His father had something to tell him. Hand in hand, they were going for a walk during which, in the manner they’d recently adopted, the father would attempt to impart to his son some lesson about life. On this occasion, the story concerned an incident that had happened to the father years earlier, on the streets of the city of Freiberg, the boy’s birthplace. The father, then a young man, had been walking along minding his own business when a stranger came up to him and in one swift motion knocked his new fur hat off his head, called him a Jew, and told him to get off the pavement. The boy dutifully listened to his father describe this scene, and he had to wonder: So what did you do? His father answered quietly that he had simply stepped into the roadway and picked up his cap. The boy and his father then walked along in silence. The boy was considering this answer. He knew his father was trying to tell him something about how times had changed and how the treatment of Jews was better today. But that’s not what the boy was thinking. Some three decades later, when Sigmund Freud recalled this scene, he couldn’t remember whether he had been ten or twelve at the time, but the impression he’d taken away from that encounter he could still summon and summarize drily: “This struck me as unheroic conduct on the part of the big, strong man who was holding the little boy by the hand.”
An impression, anyway. By the time Freud was committing this memory to paper, he was beginning to understand that any interpretation of the encounter on that long-ago day depended as much on what the boy had wanted to hear as on what the man had been trying to say, or even on what he, by now a father several times over, wished to believe about his father or himself, or about fathers and sons—depended, that is, on the vagaries of the human thought process. Not necessarily because of that conversation with his father (though maybe so; who knows?), it was the human thought process at the most basic level that Freud had grown up to explore: the pathways of nerves along which thoughts travel as they make their way through the brain. And so successful at tracing those paths were the neuroanatomists of Freud’s generation that they seemed to have reached, as one contemporary historian of science declared, “the very threshold of mind.”
That threshold was the neuron. Freud himself sought it, and in the early 1880s, as a young neuroanatomist fresh out of medical school, he even delivered a lecture before the Vienna Psychiatric Society about his own research into the structure of the nervous system. Although his subject that day was not specifically the ultimate point of connection between the fibers from two separate nerve cells within the human brain, he allowed himself a moment’s speculation on what form such a juncture might take, though he immediately, and judiciously, added, “I know that the existing material is not sufficient for a decision on this important physiological problem.”
Over the following decade, Freud metamorphosed from complacent researcher, pursuing his intuitions in a hospital laboratory in Vienna, to insecure clinician, struggling to establish a private medical practice so he could support a family, to some uneasy and perhaps unwieldy hybrid of the two, splitting his time between the laboratory and the clinic—between the theory and the practice of medicine. Yet even in private practice Freud continued to monitor neuroanatomical research as it raced toward its seemingly inevitable conclusion. If each central nerve cell in the brain exists in isolation from every other central nerve cell, as researchers had determined within Freud’s lifetime, it must still establish a connection with other central nerve cells. So: Where was it? Find that specific point of connection, as the neuroanatomists of Freud’s generation understood, and you’ll have found, at long last, the final piece in the puzzle of man.
As recently as the first quarter of the nineteenth century, that puzzle had seemed potentially insoluble, in large part because of the limitations inherent in the only instrument that might conceivably assist such an investigation. Back in the 1670s, when Antonius von Leeuwenhoek and other natural philosophers began reporting what they could see by examining terrestrial objects through a microscope, this new method of investigation might have seemed to offer limitless possibilities for anatomists. That promise, however, pretty much vanished once they got a good look at the infinitesimal scale of what they’d be studying. Leeuwenhoek himself reported that the finest pieces of matter he could see in animal tissue were simply “globules,” and for well over a century anatomists were left to concoct hypotheses about what these globulous objects might be without being able to see them any better than Leeuwenhoek had. As late as 1821, the English surgeon Charles Bell—who himself had only just recently distinguished between those nerves that carry the sensory impulses, or sensations, to the brain and those that carry the motor impulses, or instructions on how the body should respond, from the brain—gave up on the brain itself: “The endless confusion of the subject induces the physician, instead of taking the nervous system as the secure ground of his practice, to dismiss it from his course of study, as a subject presenting too great irregularity for legitimate investigation or reliance.”
Only five years later, however, British physicist Joseph Jackson Lister effectively revolutionized the microscope through improvements to the objective lens—the one nearer the specimen—that mostly eliminated distortions and color aberrations. Before this advance, neuroanatomy hadn’t been much more than speculation supported by inadequate, incomplete or imprecise observation—supported by further speculation even. Now a new breed of anatomist could explore tissue at a level of detail that was literally microscopic—the technique that would become known as histology.
In 1827, only a year after inventing his achromatic microscope, Lister himself (along with the physician Thomas Hodgkin) decisively refuted all the variations on Leeuwenhoek’s “globular” hypothesis that had arisen over the preceding century and a half. The globules, it turned out, were illusions, mere tricks of the light that Lister’s new lens system could now correct. By utilizing the achromatic microscope to examine brain tissue, Lister reported, “one sees instead of globules a multitude of very small particles, which are most irregular in shape and size.” To these particles anatomists applied a term that the first microscopists back in the seventeenth century had used to describe some of the smallest features they could see, though now it came to refer only to the basic units of organisms, both plant and animals: cells. These cells, moreover, seemed always to be accompanied by long strands, or fibers. That a relationship between these cells and fibers existed in the central nervous system—the brain and spinal cord—formed the basis of Theodor Schwann’s cell theory of 1839, a tremendous advance in knowledge from even fifteen years earlier. “However,” as one researcher reported in 1842, “the arrangement of those parts in relation to each other is still completely unknown.”
Some researchers argued that the fibers merely encircled the cells without having an anatomical connection to them. Some argued that the fibers actually emanated from the cells. Such was the density of the mass of material in the central nervous system, however, that even the achromatic microscope couldn’t penetrate it sensibly. Despite the promise of clarity that Lister’s improvements at first had seemed to offer, by the 1850s anatomists were beginning to resign themselves to the realization that the precise nature of the relationship between cells and fibers would have to remain a mystery, unless another technological advance came along.
It did, in 1858, two years after Freud was born, when the German histologist Joseph von Gerlach invented microscopic staining—a way to dye a sample so that the object under observation would bloom into a rich color against the background. The object under observation in this case was a central nerve cell, complete with an attendant network of fibers. Now neuroanatomists could see for themselves that the fibers and the cells were indeed anatomically connected. They could also see that the central nerve cells exist only in the gray matter of the brain and spinal cord, never the white. And they could see that even where a central nerve cell is part of a dense concentration within the gray matter, it exists apart from any other central nerve cell, in seeming isolation.
But if the cells themselves don’t come into contact with other cells, then how do they communicate with one another, as they clearly must? How does one cell “know” what the others are doing and therefore act in concert and thereby register a sensation or commit the nervous system to a response, an action, a thought? If the answer wasn’t in the cells, those solitary hubs, then it had to be elsewhere.
And the only elsewhere there was, was the fibers. Thanks to Gerlach’s staining method, neuroanatomists now found that the fibers extend from the central nerve cells only into the white matter of the brain and spinal cord, never the gray. But there the trail went cold. The meshwork was still too intricate for anyone to trace the paths of all the fibers from a single cell to the point where the fibers terminate. And so yet another technical innovation needed to be invented, and so yet another one was. In 1873—the same year that Freud entered the University of Vienna as a medical student—Camillo Golgi, an Italian physician, developed a superior staining method that in effect isolated the fibers in the same way that Gerlach’s had isolated the cells. Even so, researchers still couldn’t find one point of connection between the fibers of two different cells. Golgi himself thought he found one in the 1880s, but his sample was inconclusive. Still, in order to do what central nerve cells do, which is pass along impulses to one another, both prevailing theory and common sense dictated that the fibers of neighboring cells must connect, somewhere.
Not until 1889 did the Spanish histologist Santiago Ramón y Cajal discover the truth: They don’t, anywhere. This, then, was the basic unit of the brain, what the German anatomist Wilhelm Waldeyer two years later would name the neuron: each central nerve cell and its own fibers, existing apart from—that is, not connecting to—any other central nerve cells and their own fibers. But if even the least wisp of a fiber—a fibril—doesn’t connect, what does it do? It contacts, Ramón y Cajal explained. It reaches out, under the excitation of an impulse, to touch the neighboring fibril or cell, and then, when the excitation has relaxed, it retracts to its previous state of isolation. “A connection with a fiber network,” Waldeyer wrote, “or an origin from such a network, does not take place.”
Although at first the “neuron doctrine,” as Waldeyer christened it, might have seemed to contradict common sense, upon reflection the idea that communication between individual neurons was not continuous but intermittent actually went a long way toward a possible explanation of several otherwise inexplicable mental phenomena, such as the isolation of ideas, the creation of new associations, the temporary inability to remember a familiar fact, the confusion of memories. These were the phenomena, anyway, that Freud had been confronting in his private practice, where he found himself listening at length to hysterics, and wondering how to represent their worries and cures within the webwork of cells and fibers he remembered from his years of neuroanatomy.
“I am so deep in the ‘Psychology for Neurologists’ that it quite consumes me, till I have to break off overworked,” Freud wrote to a friend in April 1895. “I have never been so intensely preoccupied by anything.” By now Freud had embarked on a second career. From 1873 to 1885, first as a medical student and then as a medical researcher, he’d devoted himself to an examination of the nervous system—to research in neuroanatomy. In 1886, on the eve of his thirtieth birthday, he’d opened a private practice devoted to nervous disorders and, for the first time in his life, begun seeing patients, though he continued to conduct research on the side. After the development of the neuron theory, Freud would have had every reason to believe that if anyone were in a position to unite the psychical with the physical, it was he. He’d seen both sides. He’d studied both sides, immersing himself in the peculiar logic of each for long periods of time. He’d even written some tentative outlines to this effect over the past few years, in letters to his closest friend and constant correspondent, Wilhelm Fliess, a Berlin ear, nose, and throat doctor. Not until Freud could meet with Fliess personally late in the summer of 1895, however, and the two men could convene one of their days-long “congresses,” as Freud liked to call these occasional periods of intense and inspirational professional discussion, did he see the project whole.
Freud began composing the manuscript on the train ride from Berlin back home to Vienna that September. “I am writing so little to you only because I am writing so much for you,” Freud informed Fliess by letter on September 23. Barely two weeks later, on October 8, Freud mailed a draft to Fliess—a hundred or so handwritten pages in which he attempted to explain definitively the processes of the mind by describing exhaustively the mechanism of the brain that encases it.
And a mechanism it was. “The project,” Freud wrote, in the second sentence of the manuscript, “involves two principal ideas”: in essence, and in accord with Descartes’s philosophy and Newton’s physics, motion and matter. Freud’s principal idea number I was straightforward enough: that the workings of the brain are “subject to the general laws of motion”—that matter moves immediately adjacent matter with comprehensive cause-and-effect predictability. What contributed to Freud’s sense of urgency in composing this draft, however, was that he now knew what the “matter” was: “2. That it is to be assumed that the material particles in question are the neurons.” He based this assumption, as he made explicit several pages later, on “the knowledge of neurons which has been arrived at by modern histology.”
Yet even as he was passing the manuscript along to Fliess, Freud was starting to have his doubts. “I have been alternately proud and overjoyed and ashamed and miserable—until now, after an excess of mental torment, I apathetically tell myself: it does not yet, perhaps never will, hang together,” he wrote in the accompanying letter. “I am not succeeding with the mechanical elucidation; rather, I am inclined to listen to the quiet voice which tells me that my explanations are not yet adequate.”
In the weeks to come that inner voice softened briefly, then hardened again. “During one industrious night last week,” Freud wrote to Fliess on October 20, twelve days after posting the manuscript, “the barriers suddenly lifted, the veils dropped, and everything became transparent—from the details of the neuroses to the determinants of consciousness. Everything seemed to fall into place, the cogs meshed, I had the impression that the thing now really was a machine that shortly would function on its own.” On November 8, however, he reported that after other professional commitments had forced him to put the manuscript aside, he found he couldn’t stop thinking about it—specifically, he noted with regret, that “it required a lot of revision. At that moment,” he went on, “I rebelled against my tyrant. I felt overworked, irritated, confused, and incapable of mastering it all. So I flung it all aside. If you felt called on to form an opinion of those few sheets of paper that would justify my cry of joy at my victory, I am sorry, because you must have found it difficult.” Freud added that in another two months, after he’d fulfilled his obligations, “I may be able to get the whole thing clearer.” It was not to be. Only three weeks later he wrote to Fliess, “I no longer understand the state of mind in which I hatched the psychology; cannot conceive how I could have inflicted it on you. I believe you are still too polite; to me it appears to have been a kind of madness.”