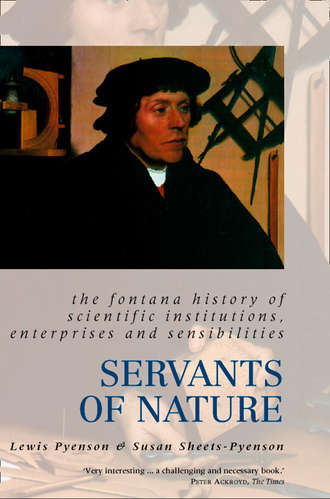
Полная версия
Servants of Nature: A History of Scientific Institutions, Enterprises and Sensibilities
Even before this reincarnation, the Académie des Sciences had begun to turn its back on its Baconian heritage, particularly the collectivist imperative. Rather than acting to generate scientific knowledge, the Académie emerged as an adjudicator, passing judgement on the merits of its members’ contributions, in pure and in applied fields. Its imprimatur became a coveted sign of national, or even international, prestige. Election to an academy seat became the crowning achievement of a life’s work; appointment to a professorial chair seemed trivial by comparison. Unlike the case during the eighteenth century, all academicians (at least in theory) were equal, since junior ranks had been abolished. By making election a process of ‘filling dead men’s shoes’ – whereby leading contenders most closely approximated the deceased (or soon to depart) academician – the Académie defined the shape of science in France. As Maurice Crosland explains, it was almost as if the subjects included in the First Class of the Institute chose the academicians.
The Académie des Sciences inaugurated new functions during the nineteenth century, such as semiannual public meetings. The Comptes rendus, created in 1835, brought the proceedings of the Academy and the eloquent éloges of its deceased members to the attention of an international community. Crosland argues that the centrality and comprehensiveness of the Comptes rendus tended to relegate all extramural efforts to oblivion. Responsibility for publications belonged to the permanent secretary, elected for life and given a comfortable annual salary of 6000 francs (about 300 pounds sterling).
By the mid nineteenth century, the Royal Society had also forsaken parts of its earlier scientific mission. Its statutes finally recognized that its role in experiment was more passive than active, more imagined than real. Regulations stated simply that the Society’s purpose was ‘to read and hear letters, reports, and other papers, concerning Philosophical matters’. In Boas Hall’s words, the atmosphere of meetings changed from ‘an atmosphere of lively discussion and debate and the frequent display of experiment’ to one that was ‘determinedly formal and lifeless’. This change did not signify that experiment was held in low regard, or that fellows had ceased to be good experimentalists, nor that nineteenth-century experiments could not be demonstrated. Rather, it indicated that the Society’s conventions had changed, placing new emphasis on results rather than processes. Papers might derive from experiment, but they were no longer accompanied by experiential demonstration.
No one disputed that experiment formed the centrepiece of the Society’s activities, only that this approach offered an imperfect and impartial view of the natural world. This was precisely the criticism of seventeenth-century natural philosophers, who believed that experimentalism offered an insufficient replacement for general principles, frameworks, and even theories. The complaint resurfaced among the spiritualists, vitalists, and theologians of later centuries, who found their concerns excluded by a materialist Royal Society. In 1878, the British geologist John Jeremiah Bigsby (1792–1881), for example, lamented the fact that in the Royal Society ‘Belief in no God and no Bible is openly paraded’. His protégé, Canadian paleobotanist John William Dawson (1820–1899), concurred that the religious scepticism of its leaders was ‘eating the heart’ out of science.
The emergence of specialized societies
Since its inception in the seventeenth century, the scientific society has sought to represent a range of philosophical interests. Sometimes art and antiquities were included to accommodate the interests of aristocratic virtuosi; certainly members’ investigations into any part of the natural sciences (and their applications) were welcomed. With the growth in size of the scientific community over the course of the eighteenth century and with the expansion of its interests, organizations devoted to the sciences in general no longer commanded attention. Scientists began to occupy themselves with a more restricted range of human experience, seeking, as well, to associate themselves with others who held similar concerns. As a result, the specialist society – one based on what we would recognize as the contents of a particular scientific discipline – began to emerge. Organizations like the Geological Society of London, founded in 1807, became known for the camaraderie and conviviality exhibited by its members, in contrast to the stiff formality displayed in the proceedings of the Royal Society.
James McClellan sees the creation of specialist societies around the turn of the nineteenth century as an accentuation of a tradition in existence decades earlier. He admits, however, that with the foundation of the Linnean Society of London in 1788, the single-discipline society became ‘less the institutional oddity, and more the norm’. In England, the Geological Society of London (1807), Zoological Society of London (1826), Royal Astronomical Society (1831), and Chemical Society of London (1841) followed in relatively quick succession. Henceforth the tendency in scientific organization was a coalescence around disciplinary interests.
As it turned out, the partial solution to specialist interests provided in the sections of the Académie des Sciences simply meant that societies restricted to certain scientific disciplines were created, on average, about a generation later in France than in England.11 French academicians did not perceive the establishment of these societies as a threat to their hegemony, since, in their view, the Académie contained the most distinguished practitioners in any particular speciality. Academicians often accepted (with some degree of condescension) senior positions in these societies, a procedure intended to elevate the new organization’s status. Unlike the Académie, specialist societies in France acted to diffuse the study of one particular science to a wider audience.
Jealousy towards rival scientific organizations was not an unreasonable reaction on the part of established societies, particularly when new fields of knowledge were represented. The danger was that specialized societies might become associated with the vanguard, and general societies with the rearguard, of the scientific enterprise. Indeed, in the case of Paul Broca (1824–1880) and the Anthropological Society in Paris, the new society offered the means of establishing the legitimacy of the nascent social science of anthropology. The formal organization attracted attention to and supplied a power base for the discipline’s founders and promoters.
The complexity of the relationship between established national societies and new specialist ones is revealed in the interactions between the Royal Society and the Geological Society. As Joseph Banks (1743–1820), the powerful president of the Royal Society, expressed his fear about the incipient importance of the geological and other London societies: ‘these new fangled Associations will finally dismantle the Royal Society and not leave the old lady a rag to cover her’. Geologists, for their part, felt that their interests commanded little respect in the eyes of the older society. One aspirant to membership was cautioned that ‘unless a geological paper be of high merit it does not meet in the Royal Society such acceptance as one in terrestrial magnetism, electricity, [or] chemistry’.
It is hardly surprising that the Royal Society should have felt some jealousy towards its younger, more lively sibling. Since its foundation, the Geological Society grew more fashionable and scientifically significant. It was composed, wrote the distinguished Cambridge geologist Adam Sedgwick (1785–1873), of ‘robust, joyous, and independent spirits, who toiled well in the field, and who did battle and cuffed opinions with much spirit and great good will’. Charles Babbage (1792–1871) lauded the Geological Society in his generally gloomy treatise on the decline of science in England, and no important geologist refused to join the organization. Furthermore, governments and universities referred geological matters not to the Royal Society but to influential members of the Geological Society. The rolls of the Society listed distinguished fellows by the 1830s – peers, members of parliament, landowners, and bankers; both Charles Darwin and the comparative anatomist Richard Owen (1804–1892) joined during that decade. Leading scientists filled positions on its Council: Roderick Murchison (1792–1871), Charles Lyell (1797–1875), and William Whewell (1794–1866) served as president; secretaries included Henry De la Beche (1796–1855) and Darwin.
At the same time that they inspired others to copy them and as they accommodated their hegemony to specialist interests, scientific bodies also fuelled petty feuds and disputes, particularly from those who had been excluded. Sometimes the jealousy remained merely isolated, negative, and remote; on other occasions, it assumed a more positive role, by uniting the dissatisfied and bringing them together to form rival institutions. Even the Canadian Sir William Dawson, whose interests had been badly served by establishment science, so esteemed the Royal Society that he modelled Canada’s national scientific society after it. A range of alternative scientific organizations – some broadly conceived, some specialist in focus – were spawned from the late eighteenth century onwards. They generally sought to democratize the scientific enterprise and to extend the benefits of membership to a larger circle.
4 Watching: Observatories in the Middle East, China, Europe and America
On a clear summer night walk as far as you can beyond the electric colours of urban life. Leave the shimmering rivers of hot air, as they snake above pavement and monument, causing the stars to twinkle. Go to where you can smell no exhaust, hear no human noise. Go at dusk and look up as the stars come out.
What may be seen? First there is the spectrum of the sky, yellow to red to faint-green and on to indigo. There may be birds, insects, and bats. There are condensation trails from high-flying jet aircraft, rapid transits of orbiting satellites, and shooting stars. Depending on one’s eyesight and location on the globe, the night sky reveals between one thousand and two thousand points of light. Located in a narrow band among these fixed stars there are seven objects that trace cyclical patterns. Until quite recently, the accidental trajectories of these seven objects – the sun, the moon, Mercury, Venus, Mars, Jupiter, and Saturn – found a central place in many civilizations. The stars have never reliably predicted the outcome of commercial, military, or personal initiatives, but their regular movements have nevertheless had an impact on our lives.
One among the seven moving stars is of critical importance. Biological cycles of growth and renewal reflect the apparent periodical motion of the sun – the solar year. We reckon age by solar cycles, not lunar ones, even in societies where the calendar is closely tied to the moon. This is so because the moon’s periodicity will not in itself predict spring inundations or winter rains, the return of migratory birds or fishes, or the best time to plant or harvest. Periodical changes in the moon’s aspect, linked with the slower, uneven velocity of the sun’s changing position in the sky, can be made to establish a yearly calendar of twelve months (each beginning with new moons) and a rather large fraction of leftover days. Astronomical science has traditionally focused on how to take care of the fraction. Once a calendar (months with a fixed number of days each) was in place, astronomical observations could be kept reliably. Records made possible the identification of cycles for the five remaining planets, the precession of the equinoxes, and predictions of such things as solar eclipses (or the possibility of them).
The existence of a calendar must not imply that we have direct access to events noted by it. Establishing a reliable chronology of antiquity – a goal sought by Europeans since medieval times – was possibly the greatest achievement of the broader historical discipline in the nineteenth century, and this occurred following a meticulous analysis of planetary records on Babylonian clay tablets. All calendars require intercalation of some sort (ours today supplies the odd day or second to round out the apparent solar year). The corrections may follow a formula or, more empirically, a celestial observation. The advantages of a determination of days and years by first principles is apparent to any head of state. Indeed, the state has generally supported astronomical observation – perhaps even (as some interpreters of Stonehenge contend) from paleolithic times.
Until Galileo Galilei pointed his telescope skyward, the seven stars that change their relative positions in a cyclical pattern were the givens of scientific endeavour. Predicting the movement of these jewels and orbs provided an arena for mathematical virtuosity, a justification for maintaining libraries, a reason for establishing schools of advanced learning, and an excuse for international collaboration. Because the patrons of this apparatus demanded practical results in the way of reliable calendars, astronomers devoted effort toward studying persistent empirical trends, such as the precession of the vernal equinox, the change in the stars behind the sun on the first day of spring.
Patrons demanded a great deal of their star gazers. Astronomers were called upon to pronounce on occasional spectacular events, such as eclipses. Through the twentieth century, astronomers have addressed meteorology – the corruptible, sublunar domain of Aristotelian physics named after the blazing objects in the sky, meteors, that were apparently as ephemeral as the rain. Astronomers were charged with telegraphical signals and radio broadcasts. They measured fundamental physical quantities in gravimetry (the gravitational constant identified by Isaac Newton) and optics (the speed of light, first calculated by Ole Christensen Römer [1644–1710]). Occasionally they chronicled the flight of migratory birds and assembled demographical statistics. They addressed whatever depended on a sharp eye and a head for figures. Until the twentieth century, astronomers were the practical masters of the realm of numbers.
The Islamic observatory
Astronomers differed from casual stargazers in that they required a special place for making observations. Observing in a grand observatory required a team of people. They had to be ready for the right moment and hope that a cloud did not intervene. In practice, this implied a support staff of servants and some form of lodging for the observers. Understanding the data required a library and calculating devices – whether pen and paper, abacus, clay tablet, or sand table. Apprentice observers had to be trained. Instruments had to be maintained. Regular reports about celestial omens and calendars had to be produced. Four thousand years of astronomical practice are continued at today’s enormous, mountain-top research installations.
We have seen that the endowed, residential college, or madrasa, was an innovation of medieval Islam. It is also to Islamic civilization that we owe the invention of the astronomical observatory. This occurred under al-Mamûn, early in the ninth century. A great patron of learning, al-Mamûn financed major astronomical complexes at Damascus and Baghdad. These possessed modifications of the instruments mentioned by Ptolemy, including an armillary sphere of concentric circles for tracking the stars, a marble mural quadrant (a graded quarter-circle mounted on a wall) for observing the height of stars above the horizon, and a five-metre gnomon or stile. The observatories assembled a group of perhaps as many as a dozen talented astronomers, one of whom was Ptolemy’s commentator al-Farghânî (Alfraganus, fl. 850), who constructed tables, or zijes, based on observations. Astrological interest, especially as it related to solar eclipses (for which Ptolemaïc data had to be corrected), was undoubtedly the motor of al-Mamûn’s astronomical patronage.
Knowledge may naturally tend to disaggregate, pooling here and there, channelling along one or another stream, evaporating into the air. The disaggregation is present in Islamic astronomy. During the Abbasid golden age, al-Mamûn’s observatories were distinct from the learned academy at Baghdad, the Dar al-Hikmah or House of Wisdom, which had been founded by Caliph Harun al-Rashid. The academy functioned as a collector and filter of learning from all sources, east and west. Greek and Indian texts, and possibly also Hebrew ones, were recovered and translated into Arabic. Among the most notable academicians was Abu Jafar Muhammad ibn Musa al-Khwarizmi (fl. 830), author of the first Arabic text on algebra (based on both Greek and Indian sources) as well as a work on Indian numerals. Al-Khwarizmi also composed a treatise on Hindu astronomy, recalculated much of Ptolemy’s data for the seven planets, and provided tables for calculating eclipses as well as trigonometrical functions. He certainly knew about the work conducted at al-Mamûn’s observatories, especially on establishing the obliquity of the ecliptic, but he chose not to incorporate the new results.
Al-Mamûn’s observatories did not survive his reign (he died in 833), but they established a precedent for observing nature. Over the next centuries, Islamic observatories extended their programmes to all the planets. The institutions became characterized by grand instruments (sometimes surpassing in size those at European locations up to the eighteenth century) and the staff (more numerous than European staff) to manoeuvre them. Observatories acquired legal status and operated under the eye of a director. The astronomical work and instrumental innovations of the polymath Ibn Sînâ (Avicenna, 980–1037), based on observations taken early in the eleventh century at an observatory financed by the amir of Isfahan at Hamadân, followed the earlier pattern. But the institutional evolution occurred unevenly. Distinguished observers, such as al-Battânî (Albategnius, fl. 880) and Ibn Yûnus (late tenth century), seem not to have availed themselves of a permanent observing facility, even though they were much concerned with astronomical innovation. Ibn Yûnus, for example, invented something akin to the method of transversals.
European commentators have traditionally celebrated Islamic savants as transmitters of Hellenistic learning; less time has been spent detailing Islamic scientific innovation. But there is no doubt that in astronomy, Islamic observations expanded and became more sophisticated. The crucial tasks of an Islamic observatory related only to the sun and the moon. One needed to establish dates of religious observances (for the Muslim lunar calendar) and times of daily prayers, keyed to sunrise and sunset. With the accessibility of Hellenistic texts, precise measurements of the sun led to interest in anomalous motions, such as precession of the equinoxes, and eventually to concern with the five remaining planets. Indeed, programmes to observe the five smaller bodies provided a justification for the permanent endowment of an observatory. It takes about thirty years of watching the sky to document all planetary regularities, and this is the working lifetime of an astronomer. Among observatories with a long-term programme was the one founded by the late eleventh-century Seljuq sultan Jalal al-Dîn Malikshâh at Isfahan; its staff of as many as eight men included al-Khayyami, the mathematician and astronomer known for his poetry as Omar Khayyam (ca.1048–ca.1131). The astronomers at the Malikshâh Observatory were the first to emphasize to their patron that it would take thirty years to record changes in the sky; from their time forward the generational argument became an astronomical watchword.
The slow pace of institutional development reflected uncertainties about using large measuring devices. One principle has dominated astronomy since antiquity: the larger the measuring device, the more accurate the observations. During the Islamic period large azimuthal rings installed on the ground to measure points on the compass were cast in copper (notably one five metres in diameter at the early twelfth-century al-Afdal al-Bataihî Observatory in Cairo), and large mural quadrants were cut into the ground and faced in marble. The moving parts of these instruments were usually made from wood – indeed, wood was preferred to brass for mural quadrants and even sextants up to the eighteenth century. But the wood warped with time and weather, especially as the large instruments were normally open to the elements. Heavy moving parts – the arm on one of Ptolemy’s rulers, for example – had to be suspended in such a way as to minimize creep. One reason for the slow growth of early observatories is that many astronomers, among them Ibn Yûnus, actually favoured small devices – even portable ones – that could be manipulated by one observer.
The peak of Islamic observatory-building took place during the thirteenth century, and its exemplar was the one founded at the city of Marâgha, south of Tabriz in present-day Iran, by Mangû, brother of the Muslim conqueror Hulâgû. Mangû, by all accounts a convinced patron of learning, seems to have first thought about inviting the most distinguished astronomer among his new Islamic subjects, Nasîr al-Dîn al-Tusî (1201–1274), to found an observatory at Beijing or possibly the Mongol capital of Qaraqurum. Indeed, during the Mongol period there was renewed intellectual interchange between East Asia and Central Asia. Several accounts refer to an Islamic astronomer, with his instruments, visiting China at just this time, and Chinese astronomers certainly travelled west. Nasîr al-Tusî may have gone east, but he certainly supervised the construction of the Marâgha Observatory, beginning in 1259. The inspiration for the Marâgha observatory, it is reasonable to assume, was Mangû’s familiarity with the Chinese tradition of constructing a new calendar for a new sovereign. To keep his hand in traditional, Chinese star-reckoning, Mangû brought Fao Mun-Ji to Marâgha at the onset of the enterprise.
To insure the life of the observatory beyond his own reign, Mangû provided it with a waqf endowment – the first known application of applying to astronomy the mechanism for endowing madrasa and hospital. The resulting revenues financed the observatory during the reigns of subsequent rulers until the dissolution of the Mongol state about 1316. Nasir al-Tusî’s sons succeeded him in directing the observatory, and it may be that he and they were the waqf administrators. The charitable endowment allowed the observatory to become an institution for instruction in the secular, or ancient sciences – the natural sciences excluded from the madrasas. In this, too, the observatory followed the pattern of Islamic teaching hospitals.
The Marâgha Observatory had a main building surmounted by a dome, through a hole in which the sun could be observed. It included an enormous library (by one account more than 400,000 volumes) and housed terrestrial and celestial globes. Many of the observatory’s rooms were excavated caves. (Astronomical observations are often made when a star passes overhead at the zenith, and for these altitude measurements, an excavated trench with a mural quadrant is fine.) Among its instruments were a fixed armillary sphere with five rings, a mural quadrant, a solar armilla, an equinoctial ring, and a parallactic ruler. The instruments went to produce a set of zijes, the so-called Ilkhâni Tables which provided data for all seven moving stars.
Marâgha formed a precedent for Mongol astronomical patronage. In the fifteenth century, Ulugh Beg (grandson of Timûr, feared in Europe as Tamerlane) erected the most magnificent of Islamic observatories at Samarqand. He became an expert astronomer, apparently constructing his observatory around an existing madrasa. He initiated astronomical instruction at the madrasa and drew talented astronomers, notably Ghiyâth al-Dîn al-Kashî (d. 1429), to the observatory, which he endowed with a waqf. The observatory apparently survived Ulugh Beg’s reign (he was murdered by his son), settling into a slow decline over the succeeding century. The Islamic tradition of grand astronomical institutions continued into the sixteenth century, with the construction of an observatory at Istanbul under the direction of Takiyüddin al-Rasid (1526–1585). It functioned for several years before being dismantled in 1580, at the request of the sultan who founded it. The last of the great Islamic observatories came in South Asia early in the eighteenth century, courtesy of Maharaja Swai Jai Singh II.