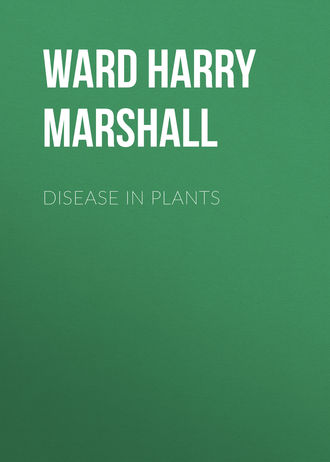
Полная версия
Disease in Plants
In this last connection a discovery made by Schulze about 1878, and followed up later by Pfeffer, Palladin, and others is of importance. Seedlings growing in the dark, or in an atmosphere devoid of carbon-dioxide in the light, become surcharged with nitrogenous bodies known as amides, formed during the breaking down of the proteids in the destructive process preceding and accompanying respiration: if the seedlings are allowed free access to light and carbon-dioxide, however, the amides disappear. The explanation is that they are combined with some of the materials of the carbohydrates, and again built up into the material of the living protoplasm.
Returning to our hypothetical starch-grain—or, rather, its parts—we have some of it retained as starch, in excess, simply because it is not needed at the moment: another portion gives up its energy in respiration, and this does work on the spot, or is lost as heat; or in the body of an organic acid, or its salt, the part in question may do lifting or pressing work by osmosis, or cause diffusion-currents from one cell to another. In the constitution of the cell-wall we may have part of our starch-grain aiding in imbibition or in the establishment of elastic tensions in turgidity: and, finally, parts may be built up into the living protoplasmic machinery of the plant.
What is true for the starch-grain is also true for any particle of salt, or water, or gas which enters into the metabolism of the living plant, regard being paid to the particular case, and circumstances in each case.
Enough has been said to show that the plant cannot be properly studied merely as the subject of chemical analysis or of physical investigation; you might as well expect to understand a watch by assays of the gold, silver, steel and diamonds of which its parts are made up, or to learn what can be got out of the proper working of a lace machine by analysing the silk put into it, and the fabric which comes out, and by taking the specific gravity of its parts and testing the physical properties of its wheels and levers.
This is not the same thing as denying the value of such knowledge, in the case of either the dead machine or the living plant: it is merely emphasising the supreme importance of the study of the structure and working of the active machinery in both cases.
Nor is it pertinent to remark on the apparent hopelessness of physiology being at present able to explain the seemingly infinite complexity of the living machinery of protoplasm and its activities. The modern locomotive is also a complex affair in its way, but it is profitable to investigate it and to know all one can of its working and possibilities, for obvious reasons: a little reflection will convince us that it is also worth while to investigate that complex machine, the plant—the working organism which alone can really enrich a country. Moreover, we ought to be encouraged by the satisfactory progress now being made, and the splendid practical results which are accruing, rather than dismayed by the prospect of unflagging labour which will be required in the future.
Enough has perhaps been said to establish the general truth that the plant is a complex machine for storing energy and material from outside, and we have seen that modern research has at least gone a long way towards determining how the living machine works.
It is hardly necessary to point out that important practical consequences may result from these phenomena of the accumulation of surplus starch or other carbohydrates in the leaves during the day, and of their disappearance during the night into the lower parts of the plant. For instance, foliage cut for fodder in the morning is far poorer in starch than if cut in the evening, and it would be very instructive to have experiments made on a large scale to test the result of feeding caterpillars or rabbits, for instance, with mulberry, vine, or other leaves in the two conditions.
Again, we now see what complications may arise if a parasitic organism gains access to the stores of carbohydrates in process of accumulation, or attacks and injures the machinery which is building up such materials, etc.
Notes to Chapter IV
The student who desires to pursue this subject further should read Sachs' Lectures, XX. and XXV., and Pfeffer's Physiology, pp. 442-566, but he will hardly arrive at the best that has been done without consulting Pfeffer's "Studien zur Energetik der Pflanzen" in the Abhandl. der Math.-Phys. Classe der Kgl. Sachss. Gesellsch. der Wiss. (Leipzig, 1892), p. 151; and Kassowitz, Allgemeine Biologie (Vienna, 1899), Bk. I., pp. 1-127.
CHAPTER V.
ROOTS AND ROOT-HAIRS
Older views as to root-hairs—Root-hairs and their development—Surface—Variations—Conditions for maximum formation—Minute structure—Adhesion to particles of soil—Functions.
On the roots of most plants are to be found delicate, silky-looking, tubular prolongations of some of the superficial cells, known as root-hairs. Malpighi (1687) seems to have been the first to observe them, and he took them for capillary tubes. Grew (1682) seems to have been responsible for the view that the roots act like sponges in taking up water.
Simon (1768) was probably the originator of the idea that these root-hairs were excretory tubules, a view that became very popular at the beginning of this century.
Meyer (1838) was perhaps the first to give a comparative account of them, and he supposed them to be delicate prolongations of the root-surface to facilitate the absorption of water.
The real importance of these organs, however, has only become apparent since Sachs, in 1859, recognised their relations to the particles of soil between which they extend and to which they cling.
In 1883 Schwarz made a very thorough study of their biological character, and in 1887 Molisch gave us new facts as to their physiology. Our knowledge of them has been rendered very much more intimate by the researches of Pfeffer and De Vries on osmotic and plasmolytic phenomena, and they serve as an excellent study of some of the best results of modern physiology.
In the normal case, such as is exemplified by a seedling wheat or bean, the root-hairs arise some distance behind the growing tip of the root, an obvious adaptation which prevents their being rubbed off by the soil, as they would be if developed on parts still actively lengthening. As those behind die off, new ones replace them in front, and so we find a wave of succession of functionally active root-hairs some little distance behind the tip of the root: the same order of events holds for each new rootlet as it emerges from the parent root, and so successive borings in the soil, made by the diverging root-tips, are thoroughly explored by these root-hairs.
Measurements have shown that in various plants the surface of root on 1 mm. of length is increased by the root-hairs in proportions given in the following table:
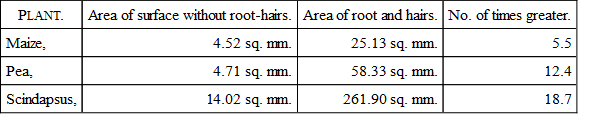
—which sufficiently establishes the general proposition that the area of the root-surface is enormously increased by these hairs.
But this does not give us any definite idea of the length of the cylinders of soil explored by these surfaces, until we find that plants such as an ordinary sunflower, hemp, or vegetable-marrow may have roots penetrating into a cubic meter of soil, in all directions, and so closely that probably no volume so large as a cubic centimeter is left unexplored. Clark found by actual measurement that the roots of a large gourd, if put end to end, extended over 25 kilometers, and Nobbe gives 520 meters for the roots of a wheat. Vetches may go nine feet deep, and oats more than three feet. The Sal, a tree of the forests of India, has roots which penetrate to a depth of 50 to 60 feet.
Some rough notion of the lengths, superficies and penetrating capacities of the roots of a large tree may be gathered from the above, but it is doubtful whether we can form any adequate ideas as to the millions of root-hairs which must be developed along the course of these subterranean boring organs.
One of the most striking results of modern enquiry into these matters, is the discovery that the number and superficial area of these root-hairs, on one and the same plant, may vary to a large extent according to the structure, as it were, of the soil, and the degree of moisture it is capable of retaining; or, with the same soil, according to the amount of water which it receives and holds. Correlations have also been observed between the development in length and surface of the rootlets themselves.
The following illustrations will suffice to show this:
Six young wheat-plants in soil kept constantly wet, developed roots the total length of which measured 365 mm. each, on the average, and almost devoid of root-hairs.
Six similar plants in soil only moderately moist, averaged 668 mm., and were well furnished (though not densely covered) with root-hairs.
Six similar plants in soil which would be termed dry, averaged 371 mm., but were densely covered with rich crops of root-hairs.
Further researches have shown that the conditions which rule the development of the root-system and root-hairs in the soil are very complex, and not always easy to trace. The most general statements we can make are the following:
There is an optimum degree of moisture in the soil which promotes the maximum development of root-hairs. If the soil is too wet they are not developed.
These facts are of importance as correlated with the ease or difficulty experienced by the roots in obtaining water, and plants such as our ordinary agricultural plants show this very distinctly.
Although, as shown in the experiments with wheat, the short roots in dry soil were more densely covered with root-hairs than the much longer roots in moderately moist soil, subsequent closer investigation shows that the total quantity and area of root-hairs is less in the former case than in the latter.
The greatest number of root-hairs are developed on roots which are growing at their best: too much moisture may prevent the formation of root-hairs: too little may induce dense growths of root-hairs locally, but the total number is reduced.
Another set of events which exerts influence on the development of root-hairs is the composition of the dilute solution—water containing dissolved salts—which surrounds them in the soil.
Thus, Schwarz found that when similar oat and wheat plants were grown with their roots in solutions of various salts, the results differed as follows:
Oats in a 15 per cent. solution of calcium chloride developed no root-hairs, though they formed in a 5 per cent. solution, and were very numerous in a 0.5 per cent. solution, or in water alone. In a 10 per cent. nutritive solution the plants developed no root-hairs, though they were abundant in a 1 per cent. solution.
Wheat plants with their roots in a 15 per cent. solution of potassium nitrate bore no root-hairs, but they were numerous in a 2 per cent. solution of the same salt.
These are extreme cases, for, although the roots were not killed, they were strongly inhibited in their growth by the more concentrated solutions. However, experiments of this kind at least bring vividly before us what variations are possible, and suggest that similar events on a smaller scale may occur in a soil which yields large quantities of soluble substances, e.g. when freshly manured. Obviously these facts have a practical significance as regards kind of soil, drainage, season (e.g. drought or wet), etc.
But there are other factors which rule the development of root-hairs, and some experiments by Lesage show that the correlations between the development of root-hairs and roots are probably much more complex than had been suspected; for he finds that if the lateral rootlets of a Bean, in a water culture, are suppressed, the main rootlet develops numerous and very long hairs to compensate the loss in surface, a matter of obvious importance in the discussion of cases where roots have been injured in the soil.
Before proceeding further it is necessary to look a little more closely into the structure of a single hair.
It is a tubular prolongation of a single cell of the external covering of the young root, usually about 1 to 3 mm. in length, and 0.01 to 0.10 mm. in diameter. In special cases the root-hairs of some water plants may reach 5 to 18 mm. in length, but of course I am referring to the ordinary land plants of agriculture and forestry. This tubular prolongation is closed and rounded off at the distal free end, and opens at the proximal end into the cell of which it is a protrusion.
The whole structure is bounded by an extremely delicate and elastic wall of cellulose, which Frank says is of special composition, almost too thin to measure in many cases, but often somewhere near 0.005 to 0.001 mm. in thickness. This thin membrane is remarkably permeable by water, or dilute solutions, as is shown by the rapidity with which a root-hair collapses if exposed to evaporation, or with which dense solutions abstract water from it, or with which solutions may be seen to penetrate it under the microscope.
Overlying the thin cell-wall proper, on the outside, is a thin gelatinous layer, a product of alteration of the outermost lamellæ of the former.
Closely lining the proper cell-wall on the inside, is an extremely thin layer of living protoplasm, and somewhere in this protoplasm is a distinct cell-nucleus.
The interior of the tube is filled with cell-sap, and it is the osmotic pressure of this cell-sap which keeps the whole living instrument tense and rigid, and the thin protoplasmic film close pressed against the cellulose cell-wall.
Nothing whatever can pass into the cell-sap, or out from it, without traversing both the lining of living protoplasm and the cell-wall.
If we gently pull a living root, of wheat, pea, mustard, etc., from a normal soil, we find particles of soil so closely adherent to the root-hairs that they cannot all be washed off without tearing the hairs: the root-hairs establish relations of contact with these particles, so close that they are cemented to the solid surfaces by means of the gelatinous layer already referred to. This peculiarity has the following consequences. In the first place, the enormous holdfast, ensured by the millions of points of adherence, enables the plant to withstand even powerful lever actions from above, and provides fixed points against which the root-tips can work as they drive deeper into the soil. In the second place, the intimate contact of the root-hairs and particles of soil, ensures that the films of water held by surface-action on the soil-particles and root-hairs shall be in continuity with the water saturating the cell-walls of the latter, and therefore with the protoplasm and cell-sap in their interior. The importance of this at periods when the soil is "dry" will be obvious, when we reflect that no soil is ever naturally so dry that surface-films of water are absent from the particles.
The fact that the root-hair contains living protoplasm, enables us to understand to a certain extent the results of the following experiments.
If we have a leafy and healthy plant, with roots, bearing numerous root-hairs, properly established in suitably moist soil in the pot, the roots cease to absorb water if the temperature of the soil falls below a certain minimum, though they recommence to do so if the temperature is raised again: this has nothing to do with the temperature of the upper parts of the plant, or of the air, and the latter may be so high that the plant rapidly droops from loss of water at the leaves, which is not being compensated owing to the inactivity of the roots.
Similarly we may have the air so cold, at a time when the soil is warm enough to keep the root-hairs actively at work, that the plant becomes surcharged with water, which escapes from the leaves like drops of dew. The temperatures necessary to cause these disturbances in the action of the living root-hairs vary for different plants, and even for different varieties of the same species.
Similar arrestation of the functions of the roots may be brought about by removing the oxygen from the soil around the root-hairs, and replacing it by carbon-dioxide, or the vapour of chloroform. If not kept too long in such a condition, the plant recovers rapidly on admitting atmospheric oxygen, which is always present in a normal well-drained soil both as gas in the capillary interspaces, and dissolved in the water on the surfaces of the particles. If the access of oxygen is delayed, however, as often happens in rainy seasons and in wet soils, the root-hairs are killed, and rot sets in. A good instance of this has lately been given by Heinricher in the case of potatoes.
Notes on Chapter V
For the further pursuit of this subject the reader should consult Sachs' Lectures, II. and XV.; Sorauer, A Popular Treatise on the Physiology of Plants, 1895, chapters II. and IV., and Pfeffer's Physiology, pp. 149-163. The principal paper on root-hairs referred to in the text is Schwarz, "Die Wurzelhaare der Pflanzen," in Unters. aus dem bot. Inst. zu Würzburg, I. Heft 2, 1883, p. 140, where a very exhaustive account of these organs will be found.
CHAPTER VI.
THE FUNCTIONS OF ROOT-HAIRS
Excretions from root-hairs—Osmotic phenomena—Turgescence—Plasmolysis—Control of the protoplasm in absorption, etc. Selective absorption.
We see then that the root-hairs are the active living instruments in absorbing the water (containing small quantities of dissolved substances) of the soil.
If the living root-hairs are so numerous and so active, however, a natural inference is that they must exert some influence on the composition or arrangement of their environment. All the teachings of modern physiology go to show that such a living cell as I have sketched cannot carry on its life, brief though it be—the root-hairs are active for about four or five days—without forming substances of the nature of excreta, and we should expect some of these to pass out to the soil.
Sachs showed, in 1860, that roots growing in contact with polished marble corrode the surface of the mineral, and Nobbe, in 1876, showed that the roots of seedlings reduce potassium permanganate, a fact which Molisch confirmed in 1887. The latter observer also proved that living root-hairs secrete substances which colour a solution of guaiacum blue, oxidise pyrogallic acid and other organic substances, and rendered it probable that they excrete some substance which inverts cane-sugar, and in some cases even small quantities of a diastatic enzyme.
Molisch also confirmed an old observation, that roots excrete carbon-dioxide; and he and Czapek showed that the root-hairs excrete acids more permanent in their nature than carbonic acid, and published a method for showing this by means of a dilute solution, slightly alkaline, of phenolphthalein.
Molisch declared that the substances secreted by root-hairs may even be observed, dissolved in drops which ooze from the surfaces of the root-hairs.
That these root-excretions, and particularly the acids, may be of service in dissolving and rendering more available various constituents of the soil is an obvious suggestion, and it is borne out by Sachs' discovery of the corrosion of marble, and by Molisch's observation that living roots slowly corrode ivory if continuously kept in contact with it.
But a deeper insight into the physiology of these organs was only possible when the meaning of the phenomena of osmosis had been rendered clearer by the researches of Pfeffer and De Vries in 1877.
De Vries showed that the turgescence of the living cell can be diminished, and even reduced to nothing, by placing the cell in contact with solutions of substances which attract water from the cell-sap: as the turgescence diminishes, the cell contracts, owing to the elasticity of the cell-wall, which was previously distended; if the abstraction of water continues, the living protoplasmic membrane lining the cell-wall contracts away from the latter. He then proved that no injury need accrue to the cell by this process of plasmolysis, since the turgescence can be restored by washing out the salt with a more dilute solution, or with pure water; and the cell may go on living and even growing as before. These phenomena can only be produced in cells where the protoplasmic lining is intact and alive.
Pfeffer showed that the whole matter depends on the properties of the living protoplasmic membrane, which, so long as it is alive, has the power of governing the entrance or exit of dissolved substances, but is as a rule freely permeable for water. If, then, substances with a powerful attraction for water are formed in the cell cavity, and of such a nature that the protoplasm does not permit their free diffusion to the exterior, they attract water, and hold it fast, and so set up the condition of hydrostatic pressure known as turgescence, the limit of which depends on the attainment of a state of equilibrium between the elastic reaction of the cell-wall and the distending power of the absorbed water. When this limit is reached, water begins to filter back again through the cell-wall. Numerous researches during the last fifteen years have shown that the sap of such a living cell as the root-hair is charged with substances of various degrees of osmotic power; bodies like sugars, amides, vegetable acids and their salts, being formed by the metabolic activity of the protoplasm and accumulated there. Moreover, we now know that the salts of the vegetable acids in particular are effective, and the researches of Warburg and Palladin in 1886 have placed it beyond reasonable doubt that these acids are continually being developed and destroyed in the living cell during normal growth and respiration, and that great variations as to quantity may be brought about by alterations in the conditions of the environment—e.g. temperature, oxygen, etc.
If, now, we bring a solution of some salt, such as potassium nitrate, which has a powerful attraction for water, on the outside of the living root-hair, the question whether the water remains in the cell, or passes out of it, merely depends on whether the substances inside or that outside have the most powerful attraction on the water in the sap, since the protoplasm allows water to pass freely.
But the protoplasmic lining may affect the whole matter in another way; for it may allow the dissolved salt, or other substance, in the solution outside or inside the cell to pass through it also, or it may take it up and fix it, or break it up or otherwise alter it.
More recent researches, and especially those of Pfeffer, have shown that these diosmotic properties of the living protoplasm are of the utmost importance in the whole matter of absorption of substances from the soil.
Let us suppose the following case. A root-hair, in full vigour, is allowed to bathe freely in a dilute solution of various substances, such as sugar, potassium nitrate, phosphates, sulphates and carbonates of iron, soda, lime, magnesium and others known by experiment to be harmless to its life.
Now it turns out to be by no means a foregone conclusion that all or any of the substances, even though freely soluble in the water, can pass through the protoplasm into the interior of the cell. Some may be allowed easy access, others may only be permitted to pass in small quantities, and others, again, may be absolutely refused access by the delicate living filter, so long as it is vigorously alive. Nor, as proved by numerous experimental cultures since De Saussure's time, is the entrance of a salt, etc., ruled by its indispensability or otherwise in the economy of the plant. And it is important to notice that only experiment can prove the point and determine which substances are absorbed and which refused by the root-hair.
If we now suppose the protoplasm to give rise to powerfully osmotic substances which accumulate in the sap-vacuole, but which are not permitted free egress through the protoplasm (and the formation of such bodies will occur if the protoplasm is actively respiring), the conditions for absorption of water, with or without any dissolved salts, which the protoplasm allows to traverse it, are set up.